Quick Look
Grade Level: 11 (10-12)
Time Required: 3 hours 15 minutes
(four to five 50-minute class periods spread over 5-6 weeks)
Expendable Cost/Group: US $30.00 This activity uses some non-expendable (reusable) items such as computers and a homemade glider launcher; see the Materials List for details.
Group Size: 3
Activity Dependency: None
Subject Areas: Measurement, Physics, Problem Solving, Science and Technology
NGSS Performance Expectations:
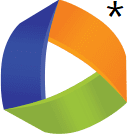
HS-ETS1-2 |
HS-ETS1-3 |
Summary
Student teams design, build and test small-sized gliders to maximize flight distance and an aerodynamic ratio, applying their knowledge of fluid dynamics to its role in flight. Students experience the entire engineering design process, from brainstorming to CAD (or by hand) drafting, including researching (physics of aerodynamics and glider components that take advantage of that science), creating materials lists, constructing, testing and evaluating—all within constraints (works with a launcher, budget limitation, maximizing flight distance to mass ratio), and concluding with a summary final report. Numerous handouts and rubrics are provided.Engineering Connection
Engineers are responsible not just for creating products, but for creating products that meet very specific criteria. For example, aeronautical engineers cannot create any flight craft that they imagine. Rather, they are presented with a set of limitations—compatibility with existing launching mechanisms, size requirements for storage and/or runway availability, design specifications such as anticipated flight time and budget considerations so the design is competitive in the market. Identifying the specifications and limitations is just the first step of the overall design process. Engineers then research the task, including known solutions for the specific problem. With this background knowledge, possible solutions are imagined, developed and then planned out more thoroughly. Prototypes of the best ideas are created, tested and evaluated for performance. If a design does not meet the criteria, the design is reimagined and the process starts anew.
Learning Objectives
After this activity, students should be able to:
- Follow the steps of the engineering design process to design a glider based on certain limitations.
- Construct and test a glider model.
- Use fluid dynamics concepts to evaluate and discuss glider performance.
Educational Standards
Each TeachEngineering lesson or activity is correlated to one or more K-12 science,
technology, engineering or math (STEM) educational standards.
All 100,000+ K-12 STEM standards covered in TeachEngineering are collected, maintained and packaged by the Achievement Standards Network (ASN),
a project of D2L (www.achievementstandards.org).
In the ASN, standards are hierarchically structured: first by source; e.g., by state; within source by type; e.g., science or mathematics;
within type by subtype, then by grade, etc.
Each TeachEngineering lesson or activity is correlated to one or more K-12 science, technology, engineering or math (STEM) educational standards.
All 100,000+ K-12 STEM standards covered in TeachEngineering are collected, maintained and packaged by the Achievement Standards Network (ASN), a project of D2L (www.achievementstandards.org).
In the ASN, standards are hierarchically structured: first by source; e.g., by state; within source by type; e.g., science or mathematics; within type by subtype, then by grade, etc.
NGSS: Next Generation Science Standards - Science
NGSS Performance Expectation | ||
---|---|---|
HS-ETS1-2. Design a solution to a complex real-world problem by breaking it down into smaller, more manageable problems that can be solved through engineering. (Grades 9 - 12) Do you agree with this alignment? |
||
Click to view other curriculum aligned to this Performance Expectation | ||
This activity focuses on the following Three Dimensional Learning aspects of NGSS: | ||
Science & Engineering Practices | Disciplinary Core Ideas | Crosscutting Concepts |
Design a solution to a complex real-world problem, based on scientific knowledge, student-generated sources of evidence, prioritized criteria, and tradeoff considerations. Alignment agreement: | Criteria may need to be broken down into simpler ones that can be approached systematically, and decisions about the priority of certain criteria over others (trade-offs) may be needed. Alignment agreement: |
NGSS Performance Expectation | ||
---|---|---|
HS-ETS1-3. Evaluate a solution to a complex real-world problem based on prioritized criteria and trade-offs that account for a range of constraints, including cost, safety, reliability, and aesthetics, as well as possible social, cultural, and environmental impacts. (Grades 9 - 12) Do you agree with this alignment? |
||
Click to view other curriculum aligned to this Performance Expectation | ||
This activity focuses on the following Three Dimensional Learning aspects of NGSS: | ||
Science & Engineering Practices | Disciplinary Core Ideas | Crosscutting Concepts |
Evaluate a solution to a complex real-world problem, based on scientific knowledge, student-generated sources of evidence, prioritized criteria, and tradeoff considerations. Alignment agreement: | When evaluating solutions it is important to take into account a range of constraints including cost, safety, reliability and aesthetics and to consider social, cultural and environmental impacts. Alignment agreement: | New technologies can have deep impacts on society and the environment, including some that were not anticipated. Analysis of costs and benefits is a critical aspect of decisions about technology. Alignment agreement: |
International Technology and Engineering Educators Association - Technology
-
Students will develop an understanding of the attributes of design.
(Grades
K -
12)
More Details
Do you agree with this alignment?
-
Students will develop abilities to apply the design process.
(Grades
K -
12)
More Details
Do you agree with this alignment?
-
Students will develop an understanding of engineering design.
(Grades
K -
12)
More Details
Do you agree with this alignment?
-
Students will develop an understanding of the role of troubleshooting, research and development, invention and innovation, and experimentation in problem solving.
(Grades
K -
12)
More Details
Do you agree with this alignment?
-
Illustrate principles, elements, and factors of design.
(Grades
9 -
12)
More Details
Do you agree with this alignment?
-
Develop a plan that incorporates knowledge from science, mathematics, and other disciplines to design or improve a technological product or system.
(Grades
9 -
12)
More Details
Do you agree with this alignment?
State Standards
Connecticut - Science
-
An object's inertia causes it to continue moving the way it is moving unless it is acted upon by a force to change its motion.
(Grade
8)
More Details
Do you agree with this alignment?
Connecticut - Technology
-
Use in depth applications of appropriate software and hardware to organize, analyze and interpret information
(Grades
9 -
12)
More Details
Do you agree with this alignment?
-
Determine a course of action that demonstrates the selection of appropriate strategies and resources for accomplishing a task, independently
(Grades
9 -
12)
More Details
Do you agree with this alignment?
Materials List
Each group needs:
- an assortment of physical materials and tools, from which to build glider models; each group determines and procures its own materials and equipment; material suggestions (not to exceed $30 per group) include foam core poster board, balsa wood, duct and electrical tape, hot and regular white glue, various types of paper, and scissors and utility knives or box cutters; tip: while students are given their choice of materials, impose a $30 budget for each team's project, giving bonus points for the least expensive prototype; it works well to provide some common materials for all groups, such as the foam core board or access to tape and glue
- computer access, either one per student or one per group
- (optional) computer-aided design program, such as Inventor, which available for free download for student use at http://www.autodesk.com/education/free-software/inventor-professional or SolidWorks (student license available for ~$150 at https://store.solidworks.com/studentstore/default.php); note: while creating a digital/CAD design is not required for the project, doing so gives students an opportunity to work with the types of programs used by present-day engineers
- (optional) Project Contract, one per student
- Glider Project Overview, one per student
- Research Evidence Handout, one per student
- Draft and Materials Handout, one per student
- (optional) Materials List Template Excel® file, one per group
- Glider Rubric, one per student
- Final Report Rubric, one per student
- pencils and paper for every student (for the pre-activity assessment)
To share with the entire class:
glider launcher, as shown in Figure 1, made according to the Glider Launcher 3D Printer Drawings —a design that requires the use of a 3D printer and the following materials, OR if no access to a 3D printer, your own glider launching device inspired by this design.
- 2 extension springs, 0.25 x 1.5-inches; available at hardware stores such as Home Depot
- 1 aluminum angle stock, 3 feet long, 0.75 x 0.0625-inches; available at hardware stores
- 5-minute, 2-part epoxy, such as http://www.homedepot.com/p/Loctite-0-47-fl-oz-Five-Minute-Instant-Mix-Epoxy-1365868/202020459
- 3D printer with PLA 3D printing material, such as https://www.amazon.com/Hatchbox-1-75mm-3D-Printer-Filament/dp/B00J0ECR5I/ref=sr_1_2?s=industrial&ie=UTF8&qid=1452691777&sr=1-2&keywords=pla+3d+printing+filament
model glider; it is recommended that you create a model glider to use in demonstrating the launching mechanism; an alternate, but less advisable, option is to obtain a pre-made glider to demonstrate the expected flight pattern, such as this 6-inch long plastic and cardboard model https://www.amazon.com/happy-deals--Camouflage-Gliders-Plastic/dp/B00F617MEA/ref=sr_1_1?ie=UTF8&qid=1452261224&sr=8-1&keywords=plastic+glider
digital scale or triple beam balance, to measure glider model masses
Worksheets and Attachments
Visit [www.teachengineering.org/activities/view/uconn_gliders_activity1] to print or download.Pre-Req Knowledge
Students should have an understanding of Newton's laws of motion, fluid statics and fluid dynamics at an Advanced Placement Physics 2 curriculum level.
Introduction/Motivation
Airplanes have become routine in our everyday lives, for long-distance shipping and personal travel, especially for those of us who live close to airports. It is easy to take for granted the existence of manned flight—which is an impressive feat of physics and engineering! How many of you know how physics enables flight? What constraints must aeronautical engineers deal with in order to meet the demands of modern flight?
Over the next few weeks, we will learn about fluid mechanics and the role that conservation of energy plays in air flow and flight. For now, let's learn about the engineering design process. (Conduct a Think-Pair-Share: What steps do engineers undergo in order to produce a product for a client?) Although many versions of the engineering design process exist, the one we will use has the following basic seven steps: 1) ask, 2) research, 3) imagine, 4) plan, 5) create, 6) test and evaluate, and 7) improve. (Write these steps on the classroom board.) Although listed in order, the process is cyclical, often folding back on itself as new challenges and solutions are realized. (Provide examples if it helps illustrate the point, using arrows to jump from one step to the next. If a student in the class has engineering experience, ask him/her to share his/her prior knowledge.)
(Write "Ask" on the board) Ask: What are the needs and constraints of the design? For this project, we will use navy aircraft as our model. (Allow time for discussion and idea sharing.) Aircraft that launch from marine aircraft carriers need to be able to fly various distances while carrying a certain payload. The aircraft is limited by the available launching mechanisms onboard as well as the military budget.
Over the next few weeks, you will work in teams and work through the remaining steps of the engineering design process to create a working model of a glider under similar needs and constraints as those just mentioned. Just as with practicing engineers, you will compete against the other teams that have been given the same requirements and constraints.
No matter what type of engineering, engineers cycle through a specific process in the design of their crafts. Imagine engineers who have been tasked by a commercial airline company to design a new type of passenger airplane. First, they need to identify the company's needs for the aircraft (how many passengers, anticipated flight capabilities and/or storage capacity; these are requirements for the design) as well as constraints (maximum size for current runway construction and/or maximum budget). With the needs and constraints clearly established, the engineers research the problem: What about the current aircraft is insufficient? What would we need in order to meet the criteria?
With the problem clearly established, the engineers imagine various solutions to the project, brainstorming many possible solutions. With multiple ideas as options, the team moves forward to plan the most-promising solution. This involves exploring more elaborate drafts and versions of the aircraft, finalizing measurements and materials. Creation of a prototype enables the engineers to test their theoretical ideas on a real model, often not at full scale. The prototype is tested and evaluated for how it aligns with the needs and constraints originally proposed by the company. Usually, the design needs improvement, which requires some redesigns to either meet the constraints or improve overall performance. Based on the complexity of the improvement, the engineering team either starts the cycle again or picks up somewhere in the middle, reworking the problem until they have an optimized solution.
Procedure
Background
Flight can be explained as being the result of several physics principles. At its fundamental level, flight requires a specific interaction of forces, as described by Newton's laws of motion. In order to accelerate forward, the forward thrust of the engines must overcome the drag forces acting on the aircraft from wind resistance. Once at altitude, aircraft can maintain a constant speed by balancing these two forces.
Aircraft are designed to minimize drag forces, which provide for a greater thrust advantage for a given engine. For the aircraft to rise, the lift provided by the wings overcomes the aircraft's weight, which depends on its mass.
The lift force is the main connection to fluid mechanics. That lift comes from a pressure differential between the top and bottom of the craft's wings. Modern wings are designed so that the wing top is highly curved, providing a greater surface area for the air to rush over. As a result, the air moves faster over the top of the wing than below it. As described by Bernoulli's principle, the difference in speed of the air around the wing causes a difference in pressure, which results in an upward force on the wing from the higher-pressure air under the wing. Thus, wing design directly impacts the ability of aircraft to fly.
Before the Activity
- Create a working launcher to use for testing the student-produced gliders. The launcher shown in Figure 1 was created with aluminum angle stock propped at a fixed angle (~30°) using 3D printed stands with a spring-loaded back piece. Build this launcher using the supplies listed in the Materials List and the Glider Launcher 3D Printer Drawings. Or, use this design as a model from which to create your own!
Figure 1. A homemade glider launcher using 3D-printed support legs and launch block. - Create (or obtain) a model glider to use in demonstrating the launching mechanism.
- Make copies of the handouts, one each per student (plus a few extra copies): Project Contract, Glider Project Overview, Research Evidence Handout, Draft and Materials Handout, Glider Rubric and Final Report Rubric.
- Have available the digital Materials List Template (Excel® file) for use by teams if desired.
- Decide on an approximate timeframe for the project, obtaining some student input as to the final deadlines to avoid major conflicts. It is recommended to allow 5-6 weeks total; see below for the recommended breakdown of time allotments for each project phase.
- In advance, have students and parents sign the Project Contract, to raise safety awareness.
- For the testing phase, identify a spacious indoor location to minimize wind interference. See the Troubleshooting Tips section for setup suggestions.
With the Students—Day 1: Introduction and Motivation
- Present to the class the Introduction/Motivation section information. Make sure each student has the necessary project handouts, including the rubrics for each project phase.
- Review the overview handout, clarify project expectations and answer any questions.
- Each team is challenged to design a glider that meets various specifications, including: launching from a standard mechanism (such as the one in Figure 1), limited to a $30 budget (with incentives to minimize the budget) and maximizing a flight distance-to-mass ratio (to simulate efficiency). As a class, review the glider rubric.
- During class on testing day, each team launches its glider from the mechanism and has its flight distance measured. Those distances will be used as part of the constructed glider evaluation as well as the ratio calculation.
- Set deadlines for each project phase, engaging students in a discussion as to what is reasonable and fair. (Note: Suggested time allotments for each project phase are provided below.)
- Organize the class into groups of one to three students each. Because much of the work for this project is done outside of classroom time, consider letting the students form their own groups.
With the Students—Research Phase: (suggested time: 1 week outside of class)
- In teams or as individuals, have students complete the research component of the project, which includes researching both the physics of aerodynamics as well as the components of gliders that take advantage of that science.
- Direct the teams to complete the required documentation as requested in the research evidence handout and submit the resulting 1-2 page summary (plus source citations) for teacher review.
With the Students—Imagine Phase: (following the completion of teacher's review of the research documentation, one 50-minute class session)
- On the board or through discussion, remind students of the project's design needs and constraints.
- Remind students that brainstorming works best when everyone contributes to an unrestrained flow of ideas.
- Have student groups brainstorm ideas for their glider designs, building on their research and previous experience.
- Monitor discussions to insure equitable and positive flow of ideas.
- Provide a three-minute warning before the end of class, prompting teams to create plans to move forward with their designs.
With the Students—Plan Phase CAD Introduction: (two, 50-minute class sessions; if software and computers are available, one per student is preferred, although one per group will suffice)
- Reserve a computer lab with computer aided design (CAD) software, such as Inventor or SolidWorks. A less ideal alternative is to project to the class a demo of the software.
- Walk students through the basics of the specific design program. The in-software tutorials are very helpful. YouTube is also a great resource for how to complete specific tasks in various CAD programs. Suggested student activities:
- creating basic sketches with smart dimensions
- extruding into three dimensions
- cutting into three-dimensional surfaces
- creating assemblies and mates
With the Students—Plan Phase: (one week outside of class; optional in-class days as time/computer availability permits)
- In teams, have students complete draft designs of their gliders using CAD software (preferred) or drawn by hand. Refer to the glider rubric to clarify upcoming testing criteria and grading.
- Direct students to create a list of materials necessary to fabricate their gliders. Expect this to include physical details and prices, as outlined in the draft and materials handout. Offer the Excel® template for team use.
- Have each team submit one "planning package" containing the two aforementioned items.
With the Students—Create Phase: (one to two weeks outside of class; optional in-class days as time permits)
- Working in their teams, have students obtain building materials, fabricate their gliders and make modifications as needed.
- Encourage students to test their gliders as they go, stressing the cyclical nature of the engineering design process. If possible, make the launching mechanism available to students for trial runs before the official testing day.
With the Students—Testing Phase: (one, 50-minute class session)
- Review the expectations for the day:
- Each group tests its glider once using the standardized launching mechanism.
- If time permits, each group tests its glider a second (and even third) time.
- The instructor marks and records each distance.
- Each team measures the mass of its glider using a digital scale or triple beam balance.
- Each team submits a rubric for evaluation of its glider.
- Review how the launching mechanism works, showing students how far back to pull the springs, which depends on the springs used in your launcher, but will be the same for each launching group. As an example, the glider launcher in Figure 1 has a pull-back of 12 cm (4.7 inches).
- After each team launches, mark where the glider first makes contact with the ground. Have students measure and record the flight distance before clearing the runway for the next group. Keep a separate record of the distances in order to evaluate the gliders by calculating the aerodynamic distance-mass ratios.
- If time permits, have students informally discuss their gliders' designs and construction.
With the Students—Evaluation and Improvement (outside of class, about three days after testing)
Have each student complete a final report, as outlined in the final report rubric and described in the Assessment section.
Assessment
Pre-Activity Assessment
Day 1 Bell Ringer: Write the following three questions on the board. As students enter the classroom, direct them to individually write their answers in their notebooks. As students are writing, circulate and read their responses, providing feedback or probing questions as appropriate. After 3-5 minutes, ask students to share their answers. Encourage students to build on each other's ideas (as engineers do in brainstorming!). Record ideas on the board for reference during the Introduction/Motivation discussion.
- What do you know about engineers?
- What do engineers do?
- How do engineers go about doing what they do?
Activity Embedded Assessment
Research Work: Each team conducts research and together develops a 1-2 page summary about the fluid mechanics of flight and how modern glider designs tie into the aerodynamics, combining their in-class learning with additional resources. They use the Research Evidence Handout for guidance and to list their source citations. Review their research to assess their comprehension and preparation for the design work.
Design & Plan: Each team submits a draft of its design idea, including a list of necessary materials and equipment, prepared according to the instructions on the Draft and Materials Handout. The draft can either be a CAD or hand-drawn sketch with measurements, angles and materials clearly labeled. The materials list includes the material type, quantity and anticipated cost based on product research, organized in the Materials List Template Excel® sheet (if desired). Each team budget is limited to $30. Review teams' "planning packages" for thoroughness and viability.
Testing for Success: Each team builds a glider that can launch from the mechanism, is sturdy enough to survive launching and flies a certain distance—criteria outlined on the Glider Rubric. Additional points are awarded for a maximized flight distance to mass ratio (to simulate flight efficiency) and minimized cost.
Post-Activity Assessment
Final Report: As a post-project analysis, have each student complete a summary final report of his or her project, as outlined in the Final Report Rubric, which they attach as the last page of the report. Expect reports to be two to four pages long, depending on how much students elaborate or the amount of space given to data tables and calculations. Make them due about three days following testing. In the reports, expect students to reflect on each project phase: revisiting the needs and constraints, discussing the aerodynamics of their individual gliders, describing and justifying any changes in their designs since submitting drafts, showing final cost calculations for the final constructed gliders, summarizing testing, providing flight distance to mass ratio calculations and explaining future design improvement ideas. Review the final reports to assess students' comprehension and mastery of the activity objectives.
Making Sense: Have students reflect about the science phenomena they explored and/or the science and engineering skills they used by completing the Making Sense Assessment.
Safety Issues
- The suggested launcher design (see Figure 1) uses springs as a source of energy. Caution students to be careful when using the springs.
- Caution students about using utility knives, epoxy glue and high-temperature and high-powered equipment during glider construction. As makes sense, provide training and/or adult supervision.
Troubleshooting Tips
To minimize wind interference, conduct testing inside. For example, place the launcher on a table in a school hallway, making sure to angle the launching mechanism so no gliders hit the ceiling. The authors used a table that was about 1 meter high and found its student gliders traveled a range of 2 to 7 meters in distance.
Activity Extensions
As an additional post-activity assessment, assign teams to prepare and give presentations to their peers about their gliders, explaining their design choices and answering audience questions. This gives each group the opportunity to "show-off" its hard work, as well as provides presentation practice.
A concluding class-wide discussion about comparing the design ideas can be very beneficial for all groups, provided the class culture can handle critical feedback. In smaller groups, have students inspect each glider and write up their own evaluative reports comparing the glider's design to its performance, discussing ideas about why the glider performed as it did.
Additional Multimedia Support
For more information on the engineering design process, including a graphic that is suitable as a student handout and classroom poster (PDF), see https://www.teachengineering.org/engrdesignprocess.php
Subscribe
Get the inside scoop on all things TeachEngineering such as new site features, curriculum updates, video releases, and more by signing up for our newsletter!Copyright
© 2016 by Regents of the University of Colorado; original © 2015 University of ConnecticutContributors
Melanie Finn-Scofield, Enrico Fermi High School, Enfield, CTSupporting Program
The Joule Fellows Program (NSF-RET), School of Engineering, University of ConnecticutAcknowledgements
This activity was developed by The Joule Fellows - Teachers in Sustainable Technologies Research Laboratories RET Program at the University of Connecticut, funded by National Science Foundation RET grant no. EEC 1403428. However, these contents do not necessarily represent the policies of the NSF, and you should not assume endorsement by the federal government.
Last modified: October 23, 2020
User Comments & Tips