Quick Look
Grade Level: 9 (9-12)
Time Required: 45 minutes
Expendable Cost/Group: US $0.20
Group Size: 2
Activity Dependency:
Subject Areas: Biology
NGSS Performance Expectations:
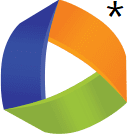
HS-LS1-1 |
Summary
Students construct paper recombinant plasmids to simulate the methods genetic engineers use to create modified bacteria. They learn what role enzymes, DNA and genes play in the modification of organisms. For the particular model they work on, they isolate a mammal insulin gene and combine it with a bacteria's gene sequence (plasmid DNA) for production of the protein insulin.Engineering Connection
Bacteria are the most common organisms modified by genetic engineers due to the simple structures of bacteria cells compared to those of eukaryotic cells. Engineers are able to add genes to bacteria using recombinant plasmids, which enable the bacteria to produce the desired beneficial proteins. Students use a paper model to simulate this real-life process used by bio-technicians.
Learning Objectives
After this activity, students should be able to:
- Model and describe the process used by engineers to modify the genome of bacteria.
- Explain why bacteria are genetically modified more often than other organisms.
- Discuss possible applications for genetically modified bacteria.
Educational Standards
Each TeachEngineering lesson or activity is correlated to one or more K-12 science,
technology, engineering or math (STEM) educational standards.
All 100,000+ K-12 STEM standards covered in TeachEngineering are collected, maintained and packaged by the Achievement Standards Network (ASN),
a project of D2L (www.achievementstandards.org).
In the ASN, standards are hierarchically structured: first by source; e.g., by state; within source by type; e.g., science or mathematics;
within type by subtype, then by grade, etc.
Each TeachEngineering lesson or activity is correlated to one or more K-12 science, technology, engineering or math (STEM) educational standards.
All 100,000+ K-12 STEM standards covered in TeachEngineering are collected, maintained and packaged by the Achievement Standards Network (ASN), a project of D2L (www.achievementstandards.org).
In the ASN, standards are hierarchically structured: first by source; e.g., by state; within source by type; e.g., science or mathematics; within type by subtype, then by grade, etc.
NGSS: Next Generation Science Standards - Science
NGSS Performance Expectation | ||
---|---|---|
HS-LS1-1. Construct an explanation based on evidence for how the structure of DNA determines the structure of proteins which carry out the essential functions of life through systems of specialized cells. (Grades 9 - 12) Do you agree with this alignment? |
||
Click to view other curriculum aligned to this Performance Expectation | ||
This activity focuses on the following Three Dimensional Learning aspects of NGSS: | ||
Science & Engineering Practices | Disciplinary Core Ideas | Crosscutting Concepts |
Construct an explanation based on valid and reliable evidence obtained from a variety of sources (including students' own investigations, models, theories, simulations, peer review) and the assumption that theories and laws that describe the natural world operate today as they did in the past and will continue to do so in the future. Alignment agreement: | Systems of specialized cells within organisms help them perform the essential functions of life. Alignment agreement: All cells contain genetic information in the form of DNA molecules. Genes are regions in the DNA that contain the instructions that code for the formation of proteins, which carry out most of the work of cells.Alignment agreement: | Investigating or designing new systems or structures requires a detailed examination of the properties of different materials, the structures of different components, and connections of components to reveal its function and/or solve a problem. Alignment agreement: |
International Technology and Engineering Educators Association - Technology
-
Medical technologies include prevention and rehabilitation, vaccines and pharmaceuticals, medical and surgical procedures, genetic engineering, and the systems within which health is protected and maintained.
(Grades
9 -
12)
More Details
Do you agree with this alignment?
-
The sciences of biochemistry and molecular biology have made it possible to manipulate the genetic information found in living creatures.
(Grades
9 -
12)
More Details
Do you agree with this alignment?
State Standards
Texas - Science
-
describe how techniques such as DNA fingerprinting, genetic modifications, and chromosomal analysis are used to study the genomes of organisms.
(Grades
9 -
11)
More Details
Do you agree with this alignment?
Materials List
Each group needs:
- scissors
- tape, 3 small pieces
- DNA Sequences for Cut-Outs, one per group; these represent plasmid DNA and mammal DNA containing the insulin gene; helpful to print page 1 on different colored paper than page 2
- Modeling Bacteria Transformation Worksheet, one per group
- Assessment Questions, one per student
- stapler (can be shared among groups)
Worksheets and Attachments
Visit [www.teachengineering.org/activities/view/uoh_genetic_lesson01_activity1] to print or download.Pre-Req Knowledge
A basic understanding of protein synthesis and DNA's role in the cell/body is helpful so students can follow how changes in DNA result in major changes in the characteristics of organisms.
Introduction/Motivation
How big are bacteria? (Answer: most are only a few micrometers. Micrometers are one millionth of a meter. 1 cm = 10,000 micrometers; 1 mm = 1,000 micrometers) Bacteria are so small that most are less than one-tenth of the diameter of a human hair.
How many different species of bacteria exist? (Answer: Estimates vary from 10 million to 1 billion species.) So far, we have only discovered less than 10,000 different species, but some scientists estimate that as many as 1 billion different species of bacteria may exist on Earth! How prolific are bacteria? Bacteria are so plentiful that a 20-ounce bottle of water may contain up to 600 million bacteria!
Bacteria are everywhere, and most of the time they are harmless. In fact, many are beneficial to people because they are useful and necessary to a healthy human body and environment. What are some examples of these "good" bacteria? (Possible answers: E.coli within the intestines of mammals, bacteria within the soil, bacteria used to make foods such as yogurt.) Without bacteria, we would not be able to digest food or produce some of our favorite foods such as yogurt and cheese. The bacteria we might generally call "bad" for us include those responsible for causing illnesses like food poisoning.
Do you think genetic engineers could use and modify bacteria for any purpose? (Answer: Yes) Bacteria are the most commonly modified organisms. Let's look at how we can modify these bacteria and why we would want to modify them.
Procedure
Background
Bacteria can be adapted to produce a number of useful materials. Because of the simple structures of bacterial cells, they are the most commonly modified organisms. Many times, and in this activity, a gene is simply added to the bacteria, causing the bacteria to be able to produce a useful protein, such as insulin. Other changes to bacteria can reconfigure the cellular respiration product to create desirable byproducts such as diesel or plastic molecules instead of the usual byproduct, such as carbon dioxide.
The common method used for genetically modifying bacteria is to use recombinant plasmids. Plasmids are circular pieces of DNA; when placed near bacteria, the plasmid is absorbed and incorporated into the bacterial cell. Once inside the bacteria, the plasmid is treated the same as the bacteria's original DNA. This means that the bacteria will use this new DNA from the plasmid to create proteins, and the plasmid will be replicated when the cell divides.
The process of creating genetically modified bacteria used in this activity is one of the simplest methods. First, a desired gene must be selected from some (any) organism, that is, the gene that codes for the creation of insulin protein, and removed from the DNA of that organism. Genes are removed using restriction enzymes. These enzymes search for specific nucleotide sequences in the DNA, called recognition sites, where they "cut" the DNA by breaking certain bonds. When the bonds are broken in a staggered manner it creates "sticky ends." If the enzyme breaks the bond to create a straight cut, the ends are called "blunt ends." The next step is to use the same restriction enzyme to cut open the plasmid.
The isolated gene is now placed where the plasmid was cut, and they are bonded together using another enzyme called ligase. Now a recombinant plasmid has been produced. The final step is to get the plasmid into a bacteria cell. Sometimes simply placing the bacteria in the correct environment is enough to artificially induce the bacteria to intake the recombinant DNA, otherwise some special method may be required if the plasmid is too large to cross the bacteria's cell membrane. Figure 1.shows a diagram of the entire process.
Before the Activity
- Gather materials and make copies of the Modeling Bacteria Transformation Worksheet, one per group, and Assessment Questions, one per person.
- Make copies of the DNA Sequences for Cut-Outs, one per group; it is helpful if the plasmid DNAs (page 1) are printed on different colored paper from the mammal DNAs (page 2) to help distinguish them during the activity. Then cut the DNA sequences into strips.
- If time is short, tape the cut-out plasmid DNA into circles with the DNA sequence facing outward. Otherwise, have students do this in step 2.
With the Students
- Divide the class into groups of two students each. Hand out to each group a worksheet and its two strips of DNA sequences, pointing out which will be used to create the plasmid, and which is the mammal DNA containing the insulin gene.
- Direct groups to tape together the ends of the plasmid DNA to form a circular piece of DNA (see Figure 2) so that the printed sequence is visible on the outside of the plasmid. This is the initial plasmid that will be modified with the insulin gene.
Figure 2. Illustration of the circular DNA created in step 2. - Find the recognition sites on both the plasmid and the mammal DNA. The restriction enzyme being used will search for a specific base pair sequence on the DNA to cut. This sequence and the cut pattern are shown in Figure 3. Have students look for this sequence on both DNA strands. Anywhere they find the full sequence is a recognition site; have them draw a dotted line at the site where the restriction enzyme will cut. Doing this usually prevents students from cutting straight across in the next step.
Figure 3. A restriction enzyme recognition site with dashed line showing where the enzyme will break apart the DNA. - Have students apply the restriction enzyme (represented by scissors in this model) to cut the DNA at the marked locations. Performed correctly, students make one cut through the plasmid and two cuts on the DNA containing the insulin gene. These cuts are made on either side of the designated gene. They are "staggered" cuts, forming the "sticky ends." Now that the desired gene is isolated, it is ready to be added to the plasmid DNA (see Figure 4).
Figure 4. In blue, the model plasmid after being cut by a restriction enzyme. In red, the mammal DNA with cuts and its isolated insulin gene with sticky ends. - Next, have students use the ligase enzyme (represented by tape in this model) to rebuild the plasmid with the new gene incorporated. If the DNA sequences have been correctly cut, the base pairs from each end of the gene match exactly with the cuts made in the plasmid. Tape the ends of the gene to the matching sticky end on the plasmid (see Figure 5).
Figure 5. The final recombinant model plasmid, a merger of the original plasmid DNA and the added insulin gene. - If groups have correctly performed their genetic engineering simulations, expect them to now have circular recombinant plasmids that each contain the gene to produce insulin. Have them staple their recombinant models on their worksheets and complete the worksheets as a team.
- Conclude the activity by administering the Assessment Questions, as described in the Assessment section. Have students staple.
Vocabulary/Definitions
DNA: Acronym for deoxyribonucleic acid, which is a molecule that contains an organism's complete genetic information.
gene: The molecular unit of an organism that contains information for a specific trait (specific DNA sequence).
genome: An entire set of genes for an organism.
model: (noun) A representation of something for imitation, comparison or analysis, sometimes on a different scale. (verb) To make something to help learn about something else that cannot be directly observed or experimented upon.
plasmid: The circular DNA structure used by bacteria.
prokaryote: A cell without a nucleus and membrane-bound organelles.
recombinant DNA: DNA to which a section has been removed and replaced (recombined) with a new sequence.
restriction enzyme: An enzyme that "cuts" DNA when specific base pair sequences are present.
Assessment
Pre-Activity Assessment
Review: Quickly do a review of bacteria, emphasizing bacteria structure. Discuss the pervasiveness of bacteria in everyday life and some roles they play, both beneficial (digestive health) and harmful (illness). Alternatively, print out the Lesson Background section and have students read through it.
Activity Embedded Assessment
Building a Model Recombinant Plasmid: Direct student pairs to apply their understanding of genetics to modify plasmids. After finishing the modification, have teams complete the Modeling Bacteria Transformation Worksheet to demonstrate their understanding of the modification process.
Post-Activity Assessment
Questions: Administer the Assessment Questions. Use the Assessment Questions Answer Key to individually gauge students' understanding of the genetic modification of bacteria and its potential benefits and dangers.
Subscribe
Get the inside scoop on all things TeachEngineering such as new site features, curriculum updates, video releases, and more by signing up for our newsletter!More Curriculum Like This

Students learn how engineers apply their understanding of DNA to manipulate specific genes to produce desired traits, and how engineers have used this practice to address current problems facing humanity. Students fill out a flow chart to list the methods to modify genes to create GMOs and example a...

As a class, students work through an example showing how DNA provides the "recipe" for making human body proteins. They see how the pattern of nucleotide bases (adenine, thymine, guanine, cytosine) forms the double helix ladder shape of DNA, and serves as the code for the steps required to make gene...

Students learn about mutations to both DNA and chromosomes, and uncontrolled changes to the genetic code. They are introduced to small-scale mutations (substitutions, deletions and insertions) and large-scale mutations (deletion duplications, inversions, insertions, translocations and nondisjunction...

Students reinforce their knowledge that DNA is the genetic material for all living things by modeling it using toothpicks and gumdrops that represent the four biochemicals (adenine, thiamine, guanine, and cytosine) that pair with each other in a specific pattern, making a double helix. Student teams...
References
Bacteria. Updated December 9, 2013. Wikipedia, The Free Encyclopedia. Accessed December 10, 2013. http://en.wikipedia.org/wiki/Bacteria
Copyright
© 2013 by Regents of the University of Colorado; original © 2013 University of HoustonContributors
Matthew Zelisko, Kimberly AndersonSupporting Program
National Science Foundation GK-12 and Research Experience for Teachers (RET) Programs, University of HoustonAcknowledgements
Acknowledgements This digital library content was developed by the University of Houston's College of Engineering under National Science Foundation GK-12 grant number DGE 0840889. However, these contents do not necessarily represent the policies of the NSF and you should not assume endorsement by the federal government.
Last modified: May 12, 2021
User Comments & Tips