Quick Look
Grade Level: 9 (8-10)
Time Required: 30 minutes
Lesson Dependency: None
Subject Areas: Biology
NGSS Performance Expectations:
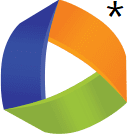
HS-LS3-2 |
Summary
Students learn about mutations to both DNA and chromosomes, and uncontrolled changes to the genetic code. They are introduced to small-scale mutations (substitutions, deletions and insertions) and large-scale mutations (deletion duplications, inversions, insertions, translocations and nondisjunctions). The effects of different mutations are studied as well as environmental factors that may increase the likelihood of mutations. Students practice their understanding of different mutation types and processes with the associated activity based off of the childhood game “telephone” . A PowerPoint® presentation and pre/post-assessments are provided.Engineering Connection
Genetic engineers are able to manipulate the genomes of organisms, however, the consequences are not always beneficial. In order to prevent harmful and unwanted mutations, it is important for engineers to understand what effects result from certain changes to organisms’ genomes (several of which can be seen by studying natural mutations) and how environmental factors can affect the probability of mutations occurring.
Learning Objectives
After this lesson, students should be able to:
- List the different types of mutations.
- Describe some possible effects of mutations.
- Explain the role of mutations in genetic syndromes.
Educational Standards
Each TeachEngineering lesson or activity is correlated to one or more K-12 science,
technology, engineering or math (STEM) educational standards.
All 100,000+ K-12 STEM standards covered in TeachEngineering are collected, maintained and packaged by the Achievement Standards Network (ASN),
a project of D2L (www.achievementstandards.org).
In the ASN, standards are hierarchically structured: first by source; e.g., by state; within source by type; e.g., science or mathematics;
within type by subtype, then by grade, etc.
Each TeachEngineering lesson or activity is correlated to one or more K-12 science, technology, engineering or math (STEM) educational standards.
All 100,000+ K-12 STEM standards covered in TeachEngineering are collected, maintained and packaged by the Achievement Standards Network (ASN), a project of D2L (www.achievementstandards.org).
In the ASN, standards are hierarchically structured: first by source; e.g., by state; within source by type; e.g., science or mathematics; within type by subtype, then by grade, etc.
NGSS: Next Generation Science Standards - Science
NGSS Performance Expectation | ||
---|---|---|
HS-LS3-2. Make and defend a claim based on evidence that inheritable genetic variations may result from: (1) new genetic combinations through meiosis, (2) viable errors occurring during replication, and/or (3) mutations caused by environmental factors. (Grades 9 - 12) Do you agree with this alignment? |
||
Click to view other curriculum aligned to this Performance Expectation | ||
This lesson focuses on the following Three Dimensional Learning aspects of NGSS: | ||
Science & Engineering Practices | Disciplinary Core Ideas | Crosscutting Concepts |
Make and defend a claim based on evidence about the natural world that reflects scientific knowledge, and student-generated evidence. Alignment agreement: | In sexual reproduction, chromosomes can sometimes swap sections during the process of meiosis (cell division), thereby creating new genetic combinations and thus more genetic variation. Although DNA replication is tightly regulated and remarkably accurate, errors do occur and result in mutations, which are also a source of genetic variation. Environmental factors can also cause mutations in genes, and viable mutations are inherited. Alignment agreement: Environmental factors also affect expression of traits, and hence affect the probability of occurrences of traits in a population. Thus the variation and distribution of traits observed depends on both genetic and environmental factors.Alignment agreement: | Empirical evidence is required to differentiate between cause and correlation and make claims about specific causes and effects. Alignment agreement: |
International Technology and Engineering Educators Association - Technology
-
The sciences of biochemistry and molecular biology have made it possible to manipulate the genetic information found in living creatures.
(Grades
9 -
12)
More Details
Do you agree with this alignment?
State Standards
Texas - Science
-
identify components of DNA, and describe how information for specifying the traits of an organism is carried in the DNA;
(Grades
9 -
11)
More Details
Do you agree with this alignment?
-
identify and illustrate changes in DNA and evaluate the significance of these changes;
(Grades
9 -
11)
More Details
Do you agree with this alignment?
Worksheets and Attachments
Visit [www.teachengineering.org/lessons/view/uoh_mutations_lesson01] to print or download.Pre-Req Knowledge
Students should have a good understanding of how DNA is copied from one cell to another through either meiosis or mitosis. They should also know that changes in the DNA or genes result in the alteration of proteins that may or may not cause noticeable changes to organisms’ traits.
Introduction/Motivation
(Be ready to show the class the 22-slide Mutations Presentation, a PowerPoint® file.)
(Slides 1-3) Introduction/Motivation: Who can tell me how Cyclops from the X-Men got his superpowers? (Answer: He’s a mutant and was born with his superpowers.) What about the Hulk? (Answer: Mutation due to exposure to gamma radiation.) And Spiderman? (Answer: Mutated when bitten by a radioactive spider.)
So, we have identified three superheroes who all gained some sort of special abilities from mutations. For Cyclops and any of the X-Men, the powers were caused by a pre-birth DNA or genome mutation. The Hulk and Spiderman powers happened a little differently since the mutations occurred later when they were exposed to radioactivity in some form or another.
Today we will discuss some of the science behind mutations. While the superpowers and abilities we just discussed may be fictional, it is true that mutations can have significant impacts on people and evidence exists that radiation exposure can lead to an increased rate of mutations. First, we will discuss the different types of mutations, then where or how they can occur. We will also talk about some environmental factors that can influence the rate of mutations, and finish by looking at some possible effects of mutations.
(Continue on, presenting the content in the Lesson Background section.)
Lesson Background and Concepts for Teachers
(Slide 4) Types of Mutations: Mutations can be classified several different ways. In this lesson, we will focus on sorting mutations by their effects on the structure of DNA or a chromosome. For this categorization, mutations can be organized into two main groups, each with multiple specific types. The two general categories are small-scale and large-scale mutations. Similar to the childhood game of "telephone" the Mutation Telephone activity helps students illustrate how mutations occur in nature.
Small-scale mutations are those that affect the DNA at the molecular level by changing the normal sequence of nucleotide base pairs. These types of mutations may occur during the process of DNA replication during either meiosis or mitosis. Three possible types of small-scale mutations may occur: substitutions, deletions and insertions.
(Slide 5) Also referred to as a “point” mutation, substitutions occur when a nucleotide is replaced with a different nucleotide in the DNA sequence. The most common substitutions involve the switching of adenine and guanine (A ↔ G) or cytosine and thymine (C ↔ T). Since the total number of nucleotides is conserved, this type of mutation only affects the codon for a single amino acid.
(Slide 6) A deletion is the removal of a nucleotide from the DNA sequence. Deletions are referred to as “frameshift” mutations because the removal of even a single nucleotide from a gene subsequently alters every codon after the mutation (it is said that the reading frame is “shifted”); this is illustrated in Figure 1 for both deletions and insertions. The change in the number of nucleotides changes which ones are normally read together.
(Slide 7) An insertion is the addition of a nucleotide to the DNA sequence. Similar to a deletion, insertions are also considered “frameshift” mutations and alter every codon that is read after the mutation.
(Slide 8) Large-scale mutations are those that affect entire portions of a chromosome. Some large-scale mutations affect only single chromosomes, others occur across nonhomologous pairs. Some large-scale mutations in the chromosome are analogous to the small-scale mutations in DNA; the difference is that for large-scale mutations, entire genes or sets of genes are altered rather than only single nucleotides of the DNA. Single chromosome mutations are most likely to occur by some error in the DNA replication stage of cell growth, and therefore could occur during meiosis or mitosis. Mutations involving multiple chromosomes are more likely to occur in meiosis during the crossing-over that occurs during the prophase I. Most of these mutations are illustrated in Figure 2.
(Slide 9) Large-scale deletion is a single chromosome mutation involving the loss of one or more gene(s) from the parent chromosome.
(Slide 10) Duplication is the addition of one or more gene(s) that are already present in the chromosome. This is a single chromosome mutation.
(Slide 11) An inversion mutation involves the complete reversal of one or more gene(s) within a chromosome. The genes are present, but the order is backwards from the parent chromosome. This is also a single chromosome mutation.
(Slide 12) Large-scale insertion involves multiple chromosomes. For this type of insertion, one or more gene(s) are removed from one chromosome and inserted into another nonhomologous chromosome. This can occur by an error during the prophase I of meiosis when the chromosomes are swapping genes to increase diversity.
(Slide 13) Translocation also involves multiple nonhomologous chromosomes. Here, the chromosomes swap one or more gene(s) with another chromosome.
(Slide 14) A nondisjunction mutation does not involve any errors in DNA replication or crossing-over. Instead, these mutations occur during the anaphase and telophase when the chromosomes are not separated correctly into the new cells. Common nondisjunctions are missing or extra chromosomes. When gametes with nondisjunctions are produced during meiosis, it can result in offspring with monosomy or trisomy (a missing or extra homologous chromosome).
(Slide 15) The effects of mutations may range from nothing to the unviability of a cell. All mutations affect the proteins that are created during protein synthesis, but not all mutations have a significant impact. The effects can also be looked at differently between the small-scale and large-scale mutations.
(Slide 16) The effects of small-scale mutations: Frameshift mutations, insertions and deletions on genes have similar effects. When a nucleotide is added or removed from the DNA sequence, the sequence is shifted and every codon after the mutation is changed, as shown in Figure 1. This results in severe alterations to the proteins that are encoded by the DNA, which can lead to a loss of functionality for those proteins.
Substitutions, or point mutations, are much more subtle and have three possible effects. The table in Figure 3 shows how some point mutations may lead to common disorders.
- Silent: The nucleotide is replaced, but the codon still produces the same amino acid.
- Missense: The codon now results in a different amino acid, which may or may not significantly alter the protein’s function.
- Nonsense: The codon now results in a “stop” command, truncating the protein at the location where the mutated codon is read; this almost always leads to a loss of protein functionality.
These mutations may occur anywhere in the DNA, so the effect of the mutation really depends on its location. If the mutation occurs in a gene, the result is an altered protein, but the mutation can also occur in a nongenic region of the DNA. In the latter case, the mutation has no effect on the organism.
(Slides 17-18) The effects of large-scale mutations are more obvious than those of small-scale mutations. Duplication of multiple genes causes those genes to be overexpressed while deletions result in missing or incomplete genes. Mutations that change the order of the genes on the chromosome—such as deletions, inversions, insertions and translocations—result in close-together genes that were previously separated either by a set of genes on the same chromosome or on another chromosome altogether. When certain genes are positioned closely together, they may encode for a “fusion protein,” which is a protein that would not normally exist but is created by a mutation in which two genes were combined. Some of these new proteins give cells a growth advantage leading to tumors and cancer. Astrocytoma, a type of brain tumor, is the result of a deletion that creates a new fusion gene that permits the cells to become cancerous.
(Slide 19) Often, large-scale mutations lead to cells that are not viable (and die due to the mutation). This is especially true with nondisjunction mutations in gametes in which entire chromosomes are missing or extra. In humans, when the gamete from a male (sperm) merges its chromosomes with the gamete from a female (egg), the offspring receive 23 chromosomes from each parent to form 23 homologous pairs, as shown in the karyotype in Figure 4. However, when one of the gametes has a nondisjunction mutation, the resulting offspring end up with only one homolog in a pair (monosomy) or with three homologs in a pair (trisomy). Most of the time, these offspring are not viable. The ones that do result in viable offspring will possess some noticeable differences due to the extra or missing chromosome; this alteration leads to a permanent syndrome in the offspring. The most well-known syndrome is trisomy 21, an extra 21st chromosome (this karyotype is shown in Figure 5); this particular nondisjunction mutation leads to Down syndrome.
(Slide 20) What can influence mutations? Mutations naturally occur over time, which is the underlying cause of evolution. As we can see, evolution is a very slow process with a net benefit to an organism, but some environmental factors may influence or induce additional mutations. These induced mutations often lead to harmful diseases, such as cancer.
Exposure to certain chemicals is one environmental factor that may induce DNA mutations. Typically, anything that we identify as carcinogenic (may cause cancer) has negative side effects on DNA, and may lead to cancer. This includes the chemicals found in cigarette smoke as well as those found in meats cooked on the grill. These chemicals belong to a larger class called mutagens, meaning they can lead to changes in genetic material.
Chemicals are not the only types of mutagens that we encounter; physical mutagens also exist in the environment, namely radiation. Ultraviolet radiation from the sun can damage genetic material by changing the properties of nucleotides in the DNA. Overexposure to ultraviolet radiation is known to lead to skin cancer. X-rays and gamma radiation are also physical mutagens and forms of ionizing radiation; this means that these types of radiation possess enough energy to remove electrons from atoms, thus forming ions and affecting how different biomolecules interact. While a typical dose of x-rays received during a medical procedure is low, it does marginally increase a person’s cancer risk.
Alternatively, retroviruses such as HIV naturally experience mutations at a much higher rate than other organisms, which can be attributed to the fact that they possess RNA instead of DNA. The process by which RNA is copied and replicated is not as precise as that of DNA. Therefore, by the time our immune system has adjusted to fight a virus like HIV, the HIV virus has already mutated again and the immune system must start over. The mutations in the HIV’s RNA lead to alterations in the protein markers on the virus that the immune system targets, and if the target is always changing, it is almost impossible for the immune system to remove the virus.
(Slides 21-22) Engineering Connection: While mutations occur naturally over time, biological engineers are able to genetically modify various organisms. Humans have been genetically modifying plants and animals for thousands of years. Humans have accomplished this by selectively breeding or inbreeding in order to produce and “improve” specific traits, such as breeding watermelons to be larger and have fewer seeds or breeding chickens to have more white meat and more breast meat.
With the advancement of technology, engineers can directly manipulate the genetic code of plants and animals. Some examples of genetically modified (and controversial) organisms include disease-resistant papaya, vitamin A-rich rice and drought-tolerant corn. Currently, researchers are studying gene editing in the womb. If it is determined that an unborn child has a disease or disability, then we may one day be able to edit the genes of the unborn child and prevent the issue from appearing in the child.
Associated Activities
- Mutation Telephone - As a way to illustrate how DNA mutations can happen, students conduct an activity similar to the childhood “telephone” game that models the biological process related to the passage of DNA from one cell to another. Then, students act as predators to test how various mutation types (normal, substitution, deletion or insertion) affect the survivability of an organism in the wild, which serves as a demonstration of natural selection based on mutation.
Vocabulary/Definitions
chromosome: A long strand of DNA wrapped around a protein that stores instructions to create several proteins. Humans have 46 chromosomes composed of 23 pairs of homologous chromosomes.
disjunction: Normal separation of chromosomes during meiosis.
DNA: A molecule that contains an organism’s complete genetic information. Abbreviation for deoxyribonucleic acid.
DNA replication: The process by which DNA is copied and passed on to new cells.
gamete: A sex cell. In mammals, the sperm and eggs. Has half the chromosomes of the parent organism.
gene: A subset of DNA that provides instructions for a cell to build a single protein.
genome: The complete genetic information for an organism; it includes all of the chromosomes.
karyotype: A picture of an organism’s genome with the chromosomes organized by homologous pairs.
meiosis: A type of cell division that occurs in sexually reproducing organisms and typically results in four cells with half the number of chromosomes of the parent. In humans, meiosis results in the creation of sperm or eggs with 23 chromosomes each.
mitosis: A type of cell division that results in two identical cells with the same number of chromosomes as the parent.
monosomy: A situation in which a homolog is missing from a chromosome pair. For example, if only one homolog exists for chromosome 21, it is called monomsomy 21.
mutagen: A physical or chemical agent that affects genetic material.
mutation: A permanent alteration in either the DNA nucleotide sequence during DNA replication or a chromosome during meiosis or mitosis.
nondisjunction: The abnormal separation of chromosomes during meiosis.
protein synthesis: A process by which the instructions contained in DNA are used to produce proteins for a cell or organism.
trisomy: A situation in which an extra chromosome is present. For example, if three homologs exist for chromosome 21, it is called trisomy 21 or Down syndrome.
Assessment
Pre-Lesson Assessment
Mutation Questions: At the beginning of class, have students write short answers to the three questions on the Pre-Lesson Worksheet. Tip: To save paper and ink, since the color of the tiger in the photograph is important for this assessment, display the worksheet via projector and have students write their answers on their own papers. Students’ answers reveal their base understanding of genetics, traits and mutations.
Lesson Summary Assessment
Mutation Questions: After the lesson, have students write short answers to the four questions on the Post-Lesson Worksheet. Tip: To save paper and ink, since the color of the tiger in the photograph is important for this assessment, display the worksheet via projector and have students write their answers on their own papers. Students’ answers reveal their comprehension of the lesson subject matter and content.
Homework
Research: Have students choose a syndrome caused by a mutation (such as extra or missing chromosomes) and write a brief, 3-5 sentence paragraph on it. Make sure they mention the specific mutation to the chromosome that leads to the syndrome and what effects that mutation causes.
Subscribe
Get the inside scoop on all things TeachEngineering such as new site features, curriculum updates, video releases, and more by signing up for our newsletter!More Curriculum Like This

Students learn how engineers apply their understanding of DNA to manipulate specific genes to produce desired traits, and how engineers have used this practice to address current problems facing humanity. Students fill out a flow chart to list the methods to modify genes to create GMOs and example a...

As a class, students work through an example showing how DNA provides the "recipe" for making human body proteins. They see how the pattern of nucleotide bases (adenine, thymine, guanine, cytosine) forms the double helix ladder shape of DNA, and serves as the code for the steps required to make gene...

Students use DNA profiling to determine who robbed a bank. After they learn how the FBI's Combined DNA Index System (CODIS) is used to match crime scene DNA with tissue sample DNA, students use CODIS principles and sample DNA fragments to determine which of three suspects matches evidence obtain at ...

Students are introduced to the latest imaging methods used to visualize molecular structures and the method of electrophoresis that is used to identify and compare genetic code (DNA).
Copyright
© 2016 by Regents of the University of Colorado; original © 2015 University of HoustonContributors
Matthew Zelisko; Kimberly Anderson; Kent KurashimaSupporting Program
National Science Foundation GK-12 and Research Experience for Teachers (RET) Programs, University of HoustonAcknowledgements
This digital library content was developed by the University of Houston's College of Engineering under National Science Foundation GK-12 grant number DGE 0840889. However, these contents do not necessarily represent the policies of the NSF and you should not assume endorsement by the federal government.
Last modified: May 27, 2019
User Comments & Tips