Quick Look
Grade Level: 6 (5-6)
Time Required: 1 hours 45 minutes
(two 50-minute class periods)
Expendable Cost/Group: US $0.40 The activity also uses some non-expendable (reusable) items; see the Materials List for details.
Group Size: 3
Activity Dependency: None
Subject Areas: Earth and Space, Measurement, Number and Operations, Physical Science, Science and Technology
NGSS Performance Expectations:
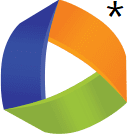
MS-ETS1-1 |
MS-ETS1-4 |
Summary
Students gain an understanding of the factors that affect wind turbine operation. Following the steps of the engineering design process, engineering teams use simple materials (cardboard and wooden dowels) to build and test their own turbine blade prototypes with the objective of maximizing electrical power output for a hypothetical situation—helping scientists power their electrical devices while doing research on a remote island. Teams explore how blade size, shape, weight and rotation interact to achieve maximal performance, and relate the power generated to energy consumed on a scale that is relevant to them in daily life. A PowerPoint® presentation, worksheet and post-activity test are provided.Engineering Connection
Mechanical engineers follow the steps of the engineering design process as they invent and evolve renewable wind energy technologies. They use their knowledge of aerodynamics, mechanics, mathematics and electricity to design and evaluate wind turbine blades and configurations. For example, engineers need to understand the mechanics of wind acting on the blades to determine the optimum blade angle. Students experience the engineering design process as they design, build, test, evaluate, redesign, re-test and re-evaluate wind turbine blades.
Learning Objectives
After this activity, students should be able to:
- Use the steps of the engineering design process to design a model wind turbine that produces energy.
- Calculate the number of wind turbines needed to power various devices.
- Identify factors that affect wind turbine performance.
- Describe how different blade configurations enhance or hinder wind turbine performance.
Educational Standards
Each TeachEngineering lesson or activity is correlated to one or more K-12 science,
technology, engineering or math (STEM) educational standards.
All 100,000+ K-12 STEM standards covered in TeachEngineering are collected, maintained and packaged by the Achievement Standards Network (ASN),
a project of D2L (www.achievementstandards.org).
In the ASN, standards are hierarchically structured: first by source; e.g., by state; within source by type; e.g., science or mathematics;
within type by subtype, then by grade, etc.
Each TeachEngineering lesson or activity is correlated to one or more K-12 science, technology, engineering or math (STEM) educational standards.
All 100,000+ K-12 STEM standards covered in TeachEngineering are collected, maintained and packaged by the Achievement Standards Network (ASN), a project of D2L (www.achievementstandards.org).
In the ASN, standards are hierarchically structured: first by source; e.g., by state; within source by type; e.g., science or mathematics; within type by subtype, then by grade, etc.
NGSS: Next Generation Science Standards - Science
NGSS Performance Expectation | ||
---|---|---|
MS-ETS1-1. Define the criteria and constraints of a design problem with sufficient precision to ensure a successful solution, taking into account relevant scientific principles and potential impacts on people and the natural environment that may limit possible solutions. (Grades 6 - 8) Do you agree with this alignment? |
||
Click to view other curriculum aligned to this Performance Expectation | ||
This activity focuses on the following Three Dimensional Learning aspects of NGSS: | ||
Science & Engineering Practices | Disciplinary Core Ideas | Crosscutting Concepts |
Define a design problem that can be solved through the development of an object, tool, process or system and includes multiple criteria and constraints, including scientific knowledge that may limit possible solutions. Alignment agreement: | The more precisely a design task's criteria and constraints can be defined, the more likely it is that the designed solution will be successful. Specification of constraints includes consideration of scientific principles and other relevant knowledge that is likely to limit possible solutions. Alignment agreement: | All human activity draws on natural resources and has both short and long-term consequences, positive as well as negative, for the health of people and the natural environment. Alignment agreement: The uses of technologies and any limitations on their use are driven by individual or societal needs, desires, and values; by the findings of scientific research; and by differences in such factors as climate, natural resources, and economic conditions.Alignment agreement: |
NGSS Performance Expectation | ||
---|---|---|
MS-ETS1-4. Develop a model to generate data for iterative testing and modification of a proposed object, tool, or process such that an optimal design can be achieved. (Grades 6 - 8) Do you agree with this alignment? |
||
Click to view other curriculum aligned to this Performance Expectation | ||
This activity focuses on the following Three Dimensional Learning aspects of NGSS: | ||
Science & Engineering Practices | Disciplinary Core Ideas | Crosscutting Concepts |
Develop a model to generate data to test ideas about designed systems, including those representing inputs and outputs. Alignment agreement: | Models of all kinds are important for testing solutions. Alignment agreement: The iterative process of testing the most promising solutions and modifying what is proposed on the basis of the test results leads to greater refinement and ultimately to an optimal solution.Alignment agreement: |
Common Core State Standards - Math
-
Understand the concept of a ratio and use ratio language to describe a ratio relationship between two quantities.
(Grade
6)
More Details
Do you agree with this alignment?
-
Compute fluently with multi-digit numbers and find common factors and multiples.
(Grade
6)
More Details
Do you agree with this alignment?
-
Apply and extend previous understandings of arithmetic to algebraic expressions.
(Grade
6)
More Details
Do you agree with this alignment?
International Technology and Engineering Educators Association - Technology
-
Apply the technology and engineering design process.
(Grades
3 -
5)
More Details
Do you agree with this alignment?
State Standards
California - Math
-
Compute fluently with multi-digit numbers and find common factors and multiples.
(Grade
6)
More Details
Do you agree with this alignment?
-
Apply and extend previous understandings of arithmetic to algebraic expressions.
(Grade
6)
More Details
Do you agree with this alignment?
-
Understand ratio concepts and use ratio reasoning to solve problems.
(Grade
6)
More Details
Do you agree with this alignment?
California - Science
-
Develop a model to generate data for iterative testing and modification of a proposed object, tool, or process such that an optimal design can be achieved.
(Grades
6 -
8)
More Details
Do you agree with this alignment?
-
Define the criteria and constraints of a design problem with sufficient precision to ensure a successful solution, taking into account relevant scientific principles and potential impacts on people and the natural environment that may limit possible solutions.
(Grades
6 -
8)
More Details
Do you agree with this alignment?
Materials List
Each group needs:
- scratch paper, 1 sheet
- scissors
- marker
- 2 sheets of cardboard, each 1 x 1 foot (30 x 30 cm)
- 2-6 wooden dowels, four-inches (10 cm) long x 3/8-inch (9.525-mm) diameter
- masking tape, 12 inches (30 cm)
- Wind Turbine Activity Worksheet, one per student
To share with the entire class:
- wind turbine tester; construct for ~$20 using materials and instructions at https://www.instructables.com/id/Model-Wind-Turbine-KidWind-Project/
- electrical multimeter
- electric fan with multiple speeds, 14-20 inch (35-50 cm) diameter preferred
- protractor
- 2 large charts, such as on a classroom board or large sheets of paper (see Table 1), into which teams record their initial design and redesign results
- computer and projector to show the Wind Turbine Design Presentation, a PowerPoint® file
Worksheets and Attachments
Visit [www.teachengineering.org/activities/view/ucd_windturbine_activity1] to print or download.Pre-Req Knowledge
A familiarity with different types of energy (such as kinetic and electrical), different forms of renewable energy (such as solar, wind, geothermal), units of measurement, and unit conversions. An understanding of current, voltage, and power is helpful.
Introduction/Motivation
Have you ever been to a tropical island? (Wait for responses.) What was it like there? Today, let’s pretend that we’re taking a trip to a deserted island in the Pacific Ocean. Close your eyes and imagine tall, rocky mountains, huge trees with canopies big enough to stretch over an entire classroom, and all sorts of animals like giant tortoises and brightly colored birds fluttering around. Recently, a group of scientists went to this island to study the wildlife, but they encountered a big problem—the island doesn’t have any electricity. Why do you think that might be a problem for the researchers? (Listen to some student responses.) With no electricity, they won’t be able to use any of their electronics like their phones, laptops, tablets and research equipment, and they will not be able to communicate with the outside world! Fortunately for them, we’re here.
The scientists are calling for engineers to help them generate power on the island renewably. What are some ways we can get renewable energy on a sunny and windy island in the Pacific? (Possible answers: Wind, solar, hydroelectric and geothermal energy sources. Wait for responses and give reasons why sources other than wind might not be the best. For example, the island does not have enough open space to put up solar panels, or the lack of sunlight during monsoon season.)
What does that leave us with? Wind energy (or wind turbines)! How do we get energy from the wind, or from wind turbines? The strong winds that pass by turn the blades of a turbine that powers an electrical generator, creating electricity.
Our job today is to design a wind turbine to generate enough electricity to power the research station on the island. To do this, each of you is going to build a model to see just how much energy we can create and which design is the best.
Procedure
Background
Wind turbines are a source of renewable energy; they turn energy from the wind, a renewable resource, into electricity. Wind is simply the movement of air, and thus has kinetic energy. As the wind blows into the blades of a turbine, its kinetic energy is transferred to the blades, which turn a rotor. The turning of the rotor powers an electrical generator, producing electricity. In this activity, the kinetic energy of the “wind” is provided by a fan. While wind turbines convert kinetic energy into electrical energy, fans do the opposite. A fan uses electrical energy to spin blades around, which move air (wind).
During this activity, students see how the movement of spinning turbine blades generates electricity by measuring the electrical output in volts and amps. The power produced by a turbine (the rate at which electrical energy is generated),
power (watts) = voltage (volts) x current (amps)
is given by the multiplication of the voltage and the current, and is measured in watts. The greater the voltage or the current, the greater the power.
Three main factors affect wind turbine power output: air density, wind speed and blade size. The greater the amount of energy captured from the wind, the greater the amount of energy that can be converted into electricity. According to Bernoulli’s principle of fluid flow (assuming constant pressure and velocity), the greater the fluid density, the greater the amount of kinetic and potential energy possessed by the fluid. Thus, the denser the air is (as is the case for colder air) the more energy the wind has. Similarly, the kinetic energy of an object,
Ekinetic = ½ m v2,
is proportional to the mass of the object (m) and the square of the object’s velocity (v). Thus, an increase in wind speed leads to an increase in the wind’s kinetic energy.
Unlike air density and wind speed, blade size does not affect the amount of energy contained by the wind, but it does affect the amount of initial energy that will be converted into electrical energy. Blade designs may be altered in many ways, including overall size, shape, material, number of blades, and blade angle. These factors affect performance mainly by manipulating the surface area and mass of the blades. The greater the blade surface area, the greater the amount of energy that is captured by the blades. Imagine holding up piece of notebook paper in a windstorm compared to holding up a large tarp—which would push you more? Conversely, the greater the mass of the blades, the more energy it takes to turn them. Furthermore, blades that extend outside the wind diameter (beyond the fan diameter) will not catch any wind and only add resistance.
Before the Activity
- Gather materials and make copies of the Wind Turbine Activity Worksheet.
- Set up the wind turbine tester.
- Place the fan so that it is about 2 feet (60 cm) away from the tester, and facing it directly.
- Write on the classroom board the seven basic steps of the engineering design process (EDP) so they are available for reference at various points throughout the activity. Alternatively, print out an 11 x 17-inch or 2 x 3-foot classroom poster showing these same Engineering Design Process Steps.
Step 1: Ask: identify the need and constraints
Step 2: Research the problem
Step 3: Imagine: develop possible solutions
Step 4: Plan: select a promising solution
Step 5: Create: build a prototype
Step 6: Test: evaluate the prototype
Step 7: Improve: redesign as needed
- Make two large class charts into which teams will record their results. As shown in Table 1, include columns for team number/name, blade design, number of blades, blade angle, and power produced. Make the blade design column big enough so teams have enough room to trace the wind turbine blade in the cell (actual size and shape). Use one chart to record data from the teams’ initial designs, and the second chart for their redesigns.
- Set up a computer and projector to show the 12-slide Wind Turbine Design Presentation, a PowerPoint® file. A script for the presentation is provided in the notes section of each slide. The slides are animated so in the slide show mode a mouse/keyboard click brings up the next text, image or slide.
With the Students: Ask, Research, Imagine, Plan, Create
- Review with students the steps of the engineering design process and emphasize how they will be following these steps to guide them to solve a unique problem, just like engineers.
- After delivering the introduction, ask the class to describe in their own words the problem that they need to solve. (EDP Step 1: Ask: identify the need and constraints)
- Ask the class what they need to do next to solve the problem. (EDP Step 2: Research the problem)
- Distribute the worksheets.
- Show students the presentation to provide an overview of the wind energy conversion, turbine mechanical components, historical information on wind turbines, and photographs of many wind turbine examples so they can pay attention to the design details, such as number of blades, blade material, blade shape, blade angle and blade attachment. Use the script provided in the slide notes.
- Direct students fill out the worksheet’s “Part 1: Introduction” section.
- Divide the class into teams of three or four students each.
- Now that they have researched the problem, it’s time for EDP Step 3: Imagine: develop possible solutions. Have students fill out the worksheet’s “Part 2: Design” section, and as a team, raise their hands when they are finished.
- Check that students have drawn and explained their designs. This completes EDP Step 4: Plan: select a promising solution. Ask students what EDP step comes next (EDP Step 5: Create: build a prototype), and let them move on to the worksheet’s “Part 3: Construction” section. Remind students that blades that extend beyond the fan diameter will not catch any wind and only add resistance.
- Give students time to construct their blades. (Suggested student construction steps are provided on the worksheet.)
- As teams finish constructing their blades, call them to the wind turbine testing station.
Testing with Each Group
- Have the students identify which step of the EDP they are at (EDP Step 6: Test: evaluate the prototype). Then, group by group, have them put their blades into the tester. Make sure the blades are evenly spaced around the rotor for the number of blades, that is, 120° apart for three blades or 90° apart for four blades.
- Let each teams select the insertion angle their blades make with the turbine, but use a protractor to make sure that all blades are at the same angle.
- Turn on the fan to the lowest setting and let the turbine get up to full speed.
- Remind students to record the voltage and amperage from the multimeter, and calculate the amount of power produced (refer to the worksheet’s “Part 4: Evaluation” section). Be sure to check their units.
- Turn the fan up to the highest setting and let the turbine to get up to full speed.
- Now that the blades are spinning at a higher rate, what differences do they observe?
- Have students record the voltage and the amperage from the multimeter, and calculate the amount of power produced. Be sure to check their units.
- Ask the students how increasing the wind speed affected the power produced by the turbine. The greater wind speed, the more energy the wind has to transfer to the turbine, which means more electricity is generated. Tell the students that we have been told that the island has a lot of strong wind, so from now on we will use the higher fan speed to model the testing conditions.
- Let students test one other angle to see which configuration produces more power. When picking another angle to test, suggest that they change the blade angle by at least 10 degrees.
- Have teams write in the class chart the results of their best runs by tracing the blade design into their team’s cell, and writing down the blade angle and amount of power produced.
- As other teams test their blades, remind students to copy into their worksheet tables the information from the class chart. While other teams are testing, have students begin the worksheet’s “Part 4: Evaluation” section, including the practice problems.
- Repeat the testing process, steps 9-21, with each of the remaining teams.
Results Evaluation with the Class > Redesign
- After all teams have tested their blades for the first time, regroup as a class to review all results. Note that this is still part of EDP Step 6: Test: evaluate the prototype(s).
- Address the worksheet’s Ultimate Questions: How much power is required to operate all of the scientists’ devices and how many turbines would it take to run them at the same time? Check that students have calculated the total power required correctly, and walk through the process if necessary. Expect the number of turbines needed to be very large (on the order of tens to hundreds of thousands). Remind the students that they are engineering model wind turbines, and that full-scale turbines generate tens to hundreds of thousands times more energy.
- Ask students if they think they are done, if they think that these results are the best they can do. Then ask what they should do next, hinting at the engineering design process (EDP Step 7: Improve: redesign as needed). To improve upon their designs, suggest they look back at their designs and their classmates’ designs to see what worked well and what did not.
- Bring attention to the various blade shapes and angles used, and how much power they produced. Ask the class to identify which team produced the most power and which produced the least. Ask if they notice any trends in how the amount of power produced correlates to blade shape, size or angle. Emphasize how engineers who design wind turbines need to balance increasing the surface area of the blades—which increases power—with increasing weight of the blades—which decreases power. Prompt the students to think of ways in which they could improve their designs.
- Direct students begin working on the worksheet’s “Part 5: Redesign” section. Instruct them to change only one variable, which might be the blade design, number of blades or blade angle.
- After teams have decided how they want to redesign their turbines, have them move on to the worksheet’s “Part 6: Re-Evaluation” section.
- Put up the second class chart to record the designs and testing results from each team’s redesigned blades.
- Let groups build and test their redesigned blade prototypes as outlined in steps 9-21.
- After all teams have tested their redesigned blades, regroup the class to review the results. Ask which teams were able to improve the amount of power produced, and what design details they altered to do so. Expect students to find that larger and wider blades at shallower angles are able to collect the most energy from the fan’s wind, somewhat contrary to what might be expected if modeling after large-scale wind turbines today that typically use three very narrow blades.
- Conclude by conducting small-group EDP reflections, assigning team design presentations and administering a post-activity test, as described in the Assessment section.
Vocabulary/Definitions
megawatt: A unit of power.
power: The rate of energy conversion.
renewable energy: Energy from resources that are continually replenished by nature, such as wind, water, sunlight, geothermal.
wind: The bulk movement of air, which contains kinetic energy.
wind turbine: A machine that converts the wind’s kinetic energy into electrical power.
windmill: A machine that uses the kinetic energy of the wind to turn blades that grind grain or perform some other work like pump water.
Assessment
Pre-Activity Assessment
Think/Pair/Share: Have students answer the following questions individually, then compare with a partner. Next, ask students to share their answers with the class.
- What is wind? (Answer: Wind is the movement of air.)
- What kind of energy does wind have? (Answer: By definition, wind has kinetic energy. It also has thermal energy since natural wind is created when air is heated up by the sun [called convection currents].)
- If you were in the middle a windstorm, which would push you more: holding up a notebook-sized piece of paper or holding up a large tarp? Why? (Answer: Holding up a large tarp would push you more because it has more surface area than a piece of paper. The larger surface area of the tarp enables it to collect more kinetic energy from the wind, which in turn would push you back with more force.)
Activity Embedded Assessment
Redesign: Before students move on to building and testing their redesigned blades, have each group present and justify its modifications. Ask the following questions:
- What are you changing about your turbine blades?
- How are you changing it?
- Why do you expect your new design to work better than the first design?
Post-Activity Assessment
EDP Reflection: After the activity, have students discuss in their teams their experiences using the steps of the engineering design process. Ask if they think engineers could solve a problem like theirs without each step of the EDP, and which step they think is the most important and why. Then ask volunteers to share with the entire class what they concluded in their groups.
Design Presentations: Have each group recap in brief presentations to the class how well their redesigned blades performed. Expect the presentations to include the following information for both the original design and the second design iteration:
- The blade shape
- The number of blades used
- The blade placement angle
- The power produced by the prototype turbine
- The number of turbines needed to power all of the scientists’ devices, as calculated in the worksheet’s “Part 4: Evaluation” section
Post-Test: After each group has presented its results, administer the four-question Wind Turbine Design Post-Test for students to complete individually. Review student answers to gauge the depth of their comprehension.
Safety Issues
- Remind students to use caution when using scissors to cut the cardboard turbine blades.
- Ensure that the turbine blades are securely fastened to the rotor before turning on the fan.
- Warn students not to stand too close to or insert their hands into the spinning turbine blades or fan.
Troubleshooting Tips
- A turbine prototype may not spin at its full potential if the blades are not correctly inserted. Make sure the blades are tightly fastened and are all at the same angle.
- When reading current and voltage measurements from a multimeter, values are likely to fluctuate since the fan and blades are not perfectly constructed or aligned. Some multimeters have a setting that averages the reading over time. Otherwise, have the instructor or students approximate the average by watching the change over time.
Activity Scaling
For higher grades, have each group test a range of values for the chosen variable of their redesigns. For example, testing blades angles from 0°-90° in steps of 15°, or testing a number of identical blades from 2-6. Then have teams plot the power output from their turbines versus their independent variables and include this as part of their presentations.
Additional Multimedia Support
BrainPOP.com has an excellent animation for introducing wind energy and wind turbines. https://www.brainpop.com/science/energy/windenergy/ (login required).
Subscribe
Get the inside scoop on all things TeachEngineering such as new site features, curriculum updates, video releases, and more by signing up for our newsletter!More Curriculum Like This

Students get introduced to the real-world technical tool of a wind turbine propeller attachment. It’s a device that efficiently harvests wind energy, and in this activity they’ll build one of their own using a LEGO wind turbine, fan, and energy meter.

Students learn how engineers harness the energy of the wind to produce power by following the engineering design process as they prototype two types of wind turbines and test to see which works best. Students also learn how engineers decide where to place wind turbines, and the advantages and disadv...

Students learn and discuss the advantages and disadvantages of renewable and non-renewable energy sources. They also learn about our nation's electric power grid and what it means for a residential home to be "off the grid."

Students learn about wind as a source of renewable energy and explore the advantages and disadvantages wind turbines and wind farms. They also learn about the effectiveness of wind turbines in varying weather conditions and how engineers work to create wind power that is cheaper, more reliable and s...
Copyright
© 2016 by Regents of the University of Colorado; original © 2016 University of California DavisContributors
Alexander KonSupporting Program
RESOURCE GK-12 Program, College of Engineering, University of California DavisAcknowledgements
This curriculum was developed by the Renewable Energy Systems Opportunity for Unified Research Collaboration and Education (RESOURCE) project in the College of Engineering under National Science Foundation GK-12 grant no. DGE 0948021. However, these contents do not necessarily represent the policies of the National Science Foundation, and you should not assume endorsement by the federal government.
A special thank you to the RESOURCE advisors, Lauren Jabusch, Alisa Lee and Jean VanderGheynst, and the RESOURCE fellows for their help in reviewing this activity. Also, many thanks to Anselmo Marin for permitting the author to test this activity in his classroom.
Last modified: July 22, 2020
User Comments & Tips