Quick Look
Grade Level: 9 (9-12)
Time Required: 1 hour
Expendable Cost/Group: US $0.00
Group Size: 4
Activity Dependency:
Subject Areas: Science and Technology
NGSS Performance Expectations:
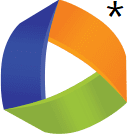
HS-ETS1-2 |
Summary
Selecting a promising solution using engineering analysis distinguishes true engineering design from "tinkering." In this activity, students are guided through an example engineering analysis scenario for a scooter. Then they perform a similar analysis on the design solutions they brainstormed in the previous activity in this unit. At activity conclusion, students should be able to defend one most-promising possible solution to their design challenge. (Note: Conduct this activity in the context of a design project that students are working on; this activity is Step 4 in a series of seven steps that guide students through the engineering design loop.)Engineering Connection
Using engineering analysis to select a promising solution is the internal guidance of a project. It can be described as the breaking down of an object, system, problem or issue into its basic elements to get at its essential features and their relationships to each other and to external elements. It is an important part of the engineering design loop that occurs many times during the completion of real-life engineering product or system design. Often, a thorough and varied analysis of a design prior to implementation leads to increased safety and efficiency in using the product.
Learning Objectives
After this activity, students should be able to:
- Describe the role of analysis in engineering.
- Evaluate alternatives using an interaction matrix analysis.
- Compare and contrast design alternatives to select the most promising idea.
Educational Standards
Each TeachEngineering lesson or activity is correlated to one or more K-12 science,
technology, engineering or math (STEM) educational standards.
All 100,000+ K-12 STEM standards covered in TeachEngineering are collected, maintained and packaged by the Achievement Standards Network (ASN),
a project of D2L (www.achievementstandards.org).
In the ASN, standards are hierarchically structured: first by source; e.g., by state; within source by type; e.g., science or mathematics;
within type by subtype, then by grade, etc.
Each TeachEngineering lesson or activity is correlated to one or more K-12 science, technology, engineering or math (STEM) educational standards.
All 100,000+ K-12 STEM standards covered in TeachEngineering are collected, maintained and packaged by the Achievement Standards Network (ASN), a project of D2L (www.achievementstandards.org).
In the ASN, standards are hierarchically structured: first by source; e.g., by state; within source by type; e.g., science or mathematics; within type by subtype, then by grade, etc.
NGSS: Next Generation Science Standards - Science
NGSS Performance Expectation | ||
---|---|---|
HS-ETS1-2. Design a solution to a complex real-world problem by breaking it down into smaller, more manageable problems that can be solved through engineering. (Grades 9 - 12) Do you agree with this alignment? |
||
Click to view other curriculum aligned to this Performance Expectation | ||
This activity focuses on the following Three Dimensional Learning aspects of NGSS: | ||
Science & Engineering Practices | Disciplinary Core Ideas | Crosscutting Concepts |
Design a solution to a complex real-world problem, based on scientific knowledge, student-generated sources of evidence, prioritized criteria, and tradeoff considerations. Alignment agreement: | Criteria may need to be broken down into simpler ones that can be approached systematically, and decisions about the priority of certain criteria over others (trade-offs) may be needed. Alignment agreement: |
Common Core State Standards - Math
-
Define appropriate quantities for the purpose of descriptive modeling.
(Grades
9 -
12)
More Details
Do you agree with this alignment?
-
Summarize, represent, and interpret data on a single count or measurement variable
(Grades
9 -
12)
More Details
Do you agree with this alignment?
International Technology and Engineering Educators Association - Technology
-
Students will develop an understanding of the attributes of design.
(Grades
K -
12)
More Details
Do you agree with this alignment?
-
Students will develop an understanding of engineering design.
(Grades
K -
12)
More Details
Do you agree with this alignment?
-
Illustrate principles, elements, and factors of design.
(Grades
9 -
12)
More Details
Do you agree with this alignment?
-
Determine the best approach by evaluating the purpose of the design.
(Grades
9 -
12)
More Details
Do you agree with this alignment?
Materials List
Each group needs
- Famous Failures Case Studies, one per student or pair of students
- Example Evaluating Alternatives Rubric
- Evaluating Alternatives Rubric
Worksheets and Attachments
Visit [www.teachengineering.org/activities/view/cub_creative_activity4] to print or download.Introduction/Motivation
Analysis is the essence of being an engineer; it is what distinguishes an engineer from a technician. Engineering analysis helps us make decisions and guide the design process. A design project without analysis is like a softball team without a coach, a ship without a sail, or a class without a teacher — imagine that! So what is engineering analysis, exactly? Basically, it is the breaking down of an object, system or problem, into its fundamental parts to understand their relationships to each other and to outside elements.
For example, let's say you are a part of a team of engineers working to reduce the number of car accidents that occur during rush-hour traffic. You might start by generating a set of design alternatives to this problem: Expand the roads and highways? Build more bike routes? Design a new subway system? Let's say your team determines the best alternative is the expansion of roads and highways. Now another design analysis is needed: How many new stoplights should be constructed? How many lanes do we need? How much money will it cost to maintain these new roads? Will many trees need to be cut down? If so, will this displace birds and other wildlife?
Do you see how the engineering analysis includes much more than the object or system being designed? Even in the case of building a new road, engineers must analyze the impacts of the new road on the city budget and the surrounding environment and impacted wildlife.
Our history has many examples of engineering projects that either succeeded or failed because of the type of engineering analysis used to evaluate the design. One "success story" in engineering is the development of modern aircraft. A century ago, the first flying machines were very unsafe. Their designs were based more on bird flight than on fundamental engineering concepts. The designers of these flying machines often tested them by jumping off great heights — sometimes meeting their death in the process.
Fortunately, over many years, engineers have developed a much better approach to engineering analysis for airplanes. Today, engineers use computer programs to design and build models of airplanes and see how the models respond to elements and forces such as weather patterns and wind shear.
Now, can anyone think of an engineering "failure?" It's hard to call an unsuccessful engineering project entirely a "failure" because we usually learn the most from failed attempts. In any case, let's take a look at some "famous failures" in engineering and see how the role of analysis played a part in the project. (Hand out the Famous Failures Case Studies to students, one per student or pair of students.)
It's important to understand that the types of engineering analysis are many and different throughout the course of every design loop, and through the course of our project development. Right now, because we are more or less in the conceptual phase of our own design challenge, we will use the engineering analysis process to help us evaluate the best design alternative from our brainstorming results. We will do this by using an "interaction matrix" in which we generate criteria for our design (attributes we think are important) and then rank each of our design alternatives according to these criteria. It may sound complicated, but it is quite useful to help guide your team's decision making process.
(Note: After conclusion of this activity, proceed to the next activity in the series, Design Steps 5 and 6: Create and Test a Prototype.)
Procedure
Background
What differentiates engineering design from simple "tinkering until you get it right" is the role of analysis in the design. Engineering analysis is the internal guidance of a project. It can be described as the breaking down of an object, system, problem or issue into its basic elements to get at its essential features and their relationships to each other and to external elements. The process of analysis is different at various stages of the design process. Toward the beginning of a project, engineers might perform an analysis to select the best design alternative. Once the best design alternative has been agreed upon, the team might perform design analyses that focus on the technical details of the design.
We can learn about the role of analysis in engineering by examining case studies of engineering projects that succeeded — and failed — due largely to the analysis used in the design. First, let's consider the development of airplanes during the past century. Many early flight pioneers died while testing their inventions. These early flying machines were based more on birds and other airborne creatures and less on fundamental engineering equations. However, these early attempts gave birth to the modern field of aeronautics and the fundamental engineering equations used to design modern airplanes.
Another major progression that has helped the aeronautic industry is the development of computer-aided design (CAD) programs. Engineers use these programs to build computer simulations of airplanes and analyze the effects of different materials, forces, weather patterns, and so on. This method of analysis is generally more accurate, cost effective, and safe than testing full-scale physical models.
Computer-aided design analysis is not confined to the aeronautic industry; many automobiles, buildings, and prosthetic devices are designed using advanced computer software.
Now, let's look at a famous engineering "failure" of our time. Some past engineering failures have been attributed to following a methodology that seemed to work. However, when scale models or forces were expanded and the designs subjected to external elements, the results were catastrophic.
The Titanic is one example. Although the Titanic was thought to be the most robust and elaborate ship of its time (in the early 1900s), it sank when its starboard side was punctured by an iceberg, causing the starboard side of the hull to fill with water and tip the giant ship. Unfortunately, the engineering analysis of the ship had been a purely static one, meaning that engineers had analyzed the ship as if it were not moving. This static analysis accounted for the weight of the passengers, cargo and wind forces, while a dynamic analysis would have taken into account external forces such as the unbalancing movement of a collision with an iceberg.
Many advanced analytical tools are needed to perform thorough engineering analyses; hence, it is often difficult for beginning design students to carry out adequate analysis. A good point to make with students is that in the "real world," engineers are continually called upon to learn and apply new engineering concepts in analysis. It is truly a lifelong learning process.
Before the Activity (Teacher Prep)
- Read and review the four attachments (case studies and answer, example rubric, blank rubric).
- Make copies of the Famous Failures Case Studies handout (one per student or pair of students), Example Evaluating Alternatives Rubric (one per team), and Evaluating Alternatives Rubric (one per team).
- Student teams should continue with the same 3-5 members each, as determined in the first activity of this unit, Design Step 1: Identify the Need.
With the Students
- To introduce the concept of engineering analysis and provide relevant examples, lead the Introduction/Motivation section with the students.
- (optional) Use the Investigating Questions to discuss the role of analysis in engineering problem solving.
- Conduct the pre-activity assessment (described in the Assessment section) to help students understand the role of analysis in engineering. This asks students to read the two Famous Failures Case Studies and answer the discussion question at the end, "What factor(s) did the engineers of both the Titanic and the Tacoma Narrows Bridge fail to include in their engineering analysis?"
- Start the main activity with the students by giving each design team a copy of the Evaluating Alternatives Rubric. (Note: This would be a good time for teams to take out the design challenge project work they have completed in previous activities [defining the problem, background research and brainstorming ideas].)
- Review the rubric instructions with the students. This is called interaction matrix analysis. It may be helpful to show and refer to the example rubric.
- Have teams begin their rubric by making lists of all the criteria they can think of to help rank their design alternatives.
- Next, have teams assess the relative importance of each criterion relative to all the other criteria.
- Have teams normalize the values by calculating each value as a proportion of a total that equals 1.
- Teams can now analyze alternative designs according to how well each design satisfies each of the identified design criteria.
- Lastly, have the teams analyze their results. The design alternative with the highest value is the "best" idea—meaning that it best meets the criteria.
Vocabulary/Definitions
computer-aided design: The use of computer technology for the design of objects; CAD design can also include symbolic information such as materials, processes, dimensions and tolerances.
dynamic analysis: An analysis of an object that accounts for interactions and uncertainties in the environment.
engineering analysis: The breaking down of an object, system or problem, into its basic parts to understand its essential features and their relationships to each other and to outside elements.
rubric: A scoring tool that lists the criteria against which to evaluate a design.
static analysis: An analysis of an object as if it was not moving.
Assessment
Pre-Activity Assessment
Famous Failures: Give each student (or pair of students) a copy of the Famous Failures Case Studies. Ask them to read the two case studies and answer the discussion question at the end: "What factor(s) did the engineers of the Titanic and the Tacoma Narrows Bridge fail to include in their engineering analysis?" See possible answers in the Famous Failures Case Studies Answers.
Activity-Embedded Assessment
Stepping through the Analysis Process: To make sure that students understand the process outlined in the Evaluating Alternatives Rubric, go through the scenario presented in the example rubric. This step-by-step example shows how a student team used the analysis process to evaluate alternatives for a scooter design.
Post-Activity Assessment
Tell It in Two Minutes: Give each team two minutes to summarize the results of the evaluating alternatives process:
- What were the team's design alternatives?
- What criteria did the team use to evaluate these alternatives?
- What was the outcome of the rubric? (In other words, which alternative received the highest score?)
- Defend why the most promising idea from the analysis is the one that should move forward in the design process.
Investigating Questions
Use the following discussion questions to help students gain understanding of an important aspect of engineering problem solving: analysis.
- What is a major difference between a technician and an engineer? (A possible answer would explain how engineers provide analysis in their design work. Engineers figure it out with careful testing, calculations and data analysis to evaluate their design.)
- What are some types of analysis that an engineer could use to test a design? (Possible answers may relate to: mathematical calculations; testing of stress, loads or function; or computer-aided analysis.)
Troubleshooting Tips
The rubric can be tricky at first. Make sure to review the process of using this matrix (and the example rubric) before asking students to complete the matrix.
Activity Extensions
Real-Life Project Analysis: As part of the teams' background research (completed in the Design Step 2: Research the Problem activity), students were asked to find examples of "real-life: engineering projects similar to their own design challenge." Now, ask students to look more closely at the analysis process used by the engineers for these projects. Did the engineers use computer simulations, build physical models, or perform another type of engineering analysis?
Additional Multimedia Support
Show students a four-minute video about the failed Tacoma Narrows Bridge including footage of the 1940 collapse, at: https://www.youtube.com/watch?v=3mclp9QmCGs.
Subscribe
Get the inside scoop on all things TeachEngineering such as new site features, curriculum updates, video releases, and more by signing up for our newsletter!More Curriculum Like This

Learn the basics of the analysis of forces engineers perform at the truss joints to calculate the strength of a truss bridge known as the “method of joints.” Find the tensions and compressions to solve systems of linear equations where the size depends on the number of elements and nodes in the trus...
References
Famous Failures of Complex Engineering Systems. December 1-5, 1997. Theoretical Foundations of Virtual Engineering and Complex Systems, AFOSR/Caltech Workshop, Control and Dynamical Systems, California Institute of Technology. Accessed January 26, 2010. (Brief recaps of Titanic sinking, Estonia ferry sinking, Tacoma Narrows Bridge collapse, Denver airport baggage handling system.)
History of Flight around the World. American Institute of Aeronautics and Astronautics. Accessed January 26, 2010. (Profiled by country and by pioneers.) https://www.aiaa.org/Secondary.aspx?id=2910
Huston, Dryver R. and Harold R. Bosch. Aerodynamic Design of Highway Structures. Winter 1996. Public Roads Magazine, Vol. 59, No. 3. Turner-Fairbank Highway Research Center, Federal Highway Administration, US Department of Transportation. Accessed January 26, 2010. http://www.tfhrc.gov/pubrds/winter96/p96w46.htm
Super Bridge: Suspension Bridges. Updated October 2000. NOVA Online, Southern Oregon Public Television. (Links to videos of Tacoma Narrows Bridge oscillation and collapse.) Accessed January 26, 2010. http://www.pbs.org/wgbh/nova/bridge/meetsusp.html
Yowell, J.L. and Carlson, D.W., Eds., Introductory Engineering Design: A Projects-Based Approach, Third Edition, Textbook for GEEN 1400: First-Year Engineering Projects, Integrated Program, College of Engineering and Applied Science, University of Colorado at Boulder, Fall 2000. Accessed April 8, 2010. http://itll.colorado.edu/index.php/courses_workshops/geen_1400/resources/textbook/
Copyright
© 2008 by Regents of the University of ColoradoContributors
Lauren Cooper; Malinda Schaefer Zarske; Denise W. CarlsonSupporting Program
Integrated Teaching and Learning Program, College of Engineering, University of Colorado BoulderAcknowledgements
The contents of this digital library curriculum were developed under a grant from the Fund for the Improvement of Postsecondary Education (FIPSE), U.S. Department of Education and National Science Foundation GK-12 grant no. 0338326. However, these contents do not necessarily represent the policies of the Department of Education or National Science Foundation, and you should not assume endorsement by the federal government.
Last modified: June 1, 2021
User Comments & Tips