Quick Look
Grade Level: 8 (6-8)
Time Required: 2 hours 30 minutes
(three 50-minute sessions)
Expendable Cost/Group: US $15.00
Group Size: 6
Activity Dependency: None
Subject Areas: Measurement, Physical Science, Physics, Problem Solving, Science and Technology
NGSS Performance Expectations:
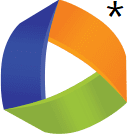
MS-ETS1-1 |
Summary
Students learn about water poverty and how water engineers can develop appropriate solutions to a problem that is plaguing nearly a sixth of the world's population. Students follow the engineering design process to design a gravity-fed water system. They choose between different system parameters such as pipe sizes, elevation differentials between entry and exit pipes, pipe lengths and tube locations to find a design that provides the maximum flow and minimum water turbidity (cloudiness) at the point of use. In this activity, students play the role of water engineers by designing and building model gravity-fed water systems, learning the key elements necessary for viable projects that help improve the lives people in developing communities.Engineering Connection
Water and hydrological engineers play a large role in addressing water poverty through the creation of appropriate technologies to provide water to developing communities. One common solution, given available topographic relief, is the implementation of gravity-fed water systems that pipe water without pumping or requiring costly energy. Typical gravity-fed system designs include a dam or spring catchment to collect the water, and the water is piped via gravity through a number of treatment processes (sedimentation, filtration, chlorination). When the water reaches the community, it is clean and in ample supply. To correctly design gravity-fed water systems requires engineers to have a good understanding of civil engineering, physics and fluid mechanics.
Learning Objectives
After this activity, students should be able to:
- Define water poverty and relate the concept to global issues and the difficulty in providing sustainable solutions.
- Relate the general physics and fluid mechanic theory and principles that allow water to flow by gravity through pipe systems.
- Design and optimize a working gravity-fed water system based on constraints.
Educational Standards
Each TeachEngineering lesson or activity is correlated to one or more K-12 science,
technology, engineering or math (STEM) educational standards.
All 100,000+ K-12 STEM standards covered in TeachEngineering are collected, maintained and packaged by the Achievement Standards Network (ASN),
a project of D2L (www.achievementstandards.org).
In the ASN, standards are hierarchically structured: first by source; e.g., by state; within source by type; e.g., science or mathematics;
within type by subtype, then by grade, etc.
Each TeachEngineering lesson or activity is correlated to one or more K-12 science, technology, engineering or math (STEM) educational standards.
All 100,000+ K-12 STEM standards covered in TeachEngineering are collected, maintained and packaged by the Achievement Standards Network (ASN), a project of D2L (www.achievementstandards.org).
In the ASN, standards are hierarchically structured: first by source; e.g., by state; within source by type; e.g., science or mathematics; within type by subtype, then by grade, etc.
NGSS: Next Generation Science Standards - Science
NGSS Performance Expectation | ||
---|---|---|
MS-ETS1-1. Define the criteria and constraints of a design problem with sufficient precision to ensure a successful solution, taking into account relevant scientific principles and potential impacts on people and the natural environment that may limit possible solutions. (Grades 6 - 8) Do you agree with this alignment? |
||
Click to view other curriculum aligned to this Performance Expectation | ||
This activity focuses on the following Three Dimensional Learning aspects of NGSS: | ||
Science & Engineering Practices | Disciplinary Core Ideas | Crosscutting Concepts |
Define a design problem that can be solved through the development of an object, tool, process or system and includes multiple criteria and constraints, including scientific knowledge that may limit possible solutions. Alignment agreement: | The more precisely a design task's criteria and constraints can be defined, the more likely it is that the designed solution will be successful. Specification of constraints includes consideration of scientific principles and other relevant knowledge that is likely to limit possible solutions. Alignment agreement: | All human activity draws on natural resources and has both short and long-term consequences, positive as well as negative, for the health of people and the natural environment. Alignment agreement: The uses of technologies and any limitations on their use are driven by individual or societal needs, desires, and values; by the findings of scientific research; and by differences in such factors as climate, natural resources, and economic conditions.Alignment agreement: |
International Technology and Engineering Educators Association - Technology
-
Apply the technology and engineering design process.
(Grades
6 -
8)
More Details
Do you agree with this alignment?
-
Create solutions to problems by identifying and applying human factors in design.
(Grades
6 -
8)
More Details
Do you agree with this alignment?
State Standards
Colorado - Science
-
Predict and evaluate the movement of an object by examining the forces applied to it
(Grade
8)
More Details
Do you agree with this alignment?
Materials List
For introductory teacher presentation:
- Gravity-Fed Water Systems Presentation (a PowerPoint® file)
- computer and LCD projector to show a PowerPoint presentation
- drill with ½" bit to start holes in tubs
- utility or Exacto® knife to further widen holes (as an alternative, ask the hardware store where you buy the tubs to drill the holes for you; ensure you have the pipe adapters to illustrate and clearly explain the layout of the holes)
Each group needs:
- access to water or, if necessary, a 5-gallon bucket filled with water
- safety glasses/goggles, one per student
- Gravity-Fed System Design Worksheet, one per student
- turbidity chart (to measure cloudiness), on the last slide of the presentation
- 2 calculators, for two students to check calculations
- 2 6-ft long 0.25" or 0.5" clear polymer tubing (or 1 of each; available at hardware stores)
- 1 3-ft long 0.5" clear polymer tubing to represent the tap (available at hardware stores)
- 5 0.25-in and 5 0.5-in threaded hose barbs (thread on one end and barbed on the other), with o-ring that goes over the threaded section, along with a metal or plastic nut that threads on to the threaded section (available at hardware stores)
- 3 clear 5-gallon tubs, holes drilled for the following; for more information, see the Procedures section and Figure 1 (tubs available at hardware/discount stores): pipe adaptors for exit of spring catchment basin or dam (tub 1), entrance and exit for sedimentation tank (tub 2), entrance only for "community" tank (tub 3)
- 2 cups of dirt (sediment)
- 2 stopwatches to measure flow rate
- (5) 2' x 2' cardboard boxes, or larger cardboard boxes (to place tanks on)
- 1 clear plastic cup (end user) (note: this is the cup of water that students fill with tub 3 water to insinuate drinking)
To share with the entire class:
- 12 6-ft long 0.25" clear polymer tubing (available at hardware stores)
- 12 6-ft long 0.5" clear polymer tubing (available at hardware stores)
- 3 hoses connected to classroom taps to fill tub 1 (dam/catchment) <
Worksheets and Attachments
Visit [www.teachengineering.org/activities/view/cub_gravityfed_activity1] to print or download.Introduction/Motivation
Have you ever missed school because you were sick with some kind of "stomach bug?" How much school did you miss? A doctor might tell you to stay hydrated by drinking plenty of water. But, imagine if it was the water that kept making you sick, and if drinking more water kept you in a cycle of sickness! What if you were too sick to return to school? What if your family could not afford the doctor bills? This is an example of what we call "water poverty." In fact, any source of water that is too dirty, too far away, or too expensive is a form of water poverty. The cycle of water poverty is experienced by more than 800 million people around the world.
The problem of water poverty has led to an uprising of engineering development projects to aid in the building of water infrastructure to provide clean water to poor and rural communities in developing countries. The typical water distribution treatment system installed in a community usually falls either within centralized systems (wells, gravity-fed systems) or point-of-use systems (POU), which is the treatment of water at the household level, either by filtration or chemical disinfection. Gravity-fed water systems—often a high initial investment due to the cost of pipes, and often a lengthy distance from the clean water source to the community—typically have a longer lifespan due to no moving parts (pumps) or energy requirements (electricity, gas, diesel). These types of water systems, therefore, are more sustainable. Since piped systems can only create a water flow by gravity from a higher to lower elevation, gravity-fed systems only work if the water source is significantly higher in elevation than the community being served.
When engineers design gravity-fed water systems, they consider many constraints (requirements and limitations) on system performance:
- Water Source Quality and Quantity: If the source water is not clean, it cannot be used. If the water quantity changes throughout the day and does not meet the community water demand, it will not work.
- Topography (how the surrounding land is shaped): The topography must allow for water to flow at all locations along the pipe through pressure differentials that result from water flowing from higher to lower elevations.
- System Demand: This is a function of the number of people using the system and the amount of water allocated to each person.
- Frictional Loss: Just like with a system that has moving parts, friction can occur in water systems as the water flows through the pipes and results in loss of pressure along the pipe, which can prevent flow altogether.
- Pipe Size and Length: Engineers design pipe size based on how much water is needed by the community and how much friction will occur through the pipes.
In addition to considering constraints before designing and building water systems, engineers seek to discover the turbidity of water before deciding to use it as a water source. Is it clean enough? Turbidity is the "cloudiness" or "darkness" of drinking water, used as a metric for contamination, whereas a higher NTU (or, Nephelometric Turbidity Units), would mean a higher turbidity, and thereby higher contamination. NTU goes up as the water becomes cloudier and/or darker. Clearly, water with high turbidity would have a higher chance for contamination
Procedure
Before the Activity
- Gather materials. Make sure you have twelve (12) 6-ft sections of both 0.25" and 0.5" polymer tubing, twenty (20) 0.25" and twenty (20) 0.5" threaded hose barbs each with o-ring and nuts, twelve (12) 5-gallon clear tubs, six (6) 3-ft sections of 0.5" polymer tubing, a bin of dirt, ten (10) clear plastic cups, twenty (20) cardboard boxes.
- Drill holes in the ends of each tub, as illustrated in Figure 1:
Tub 1: Dam or Spring Box, with two (2) exit holes positioned on the bottom of the tub, one for the 0.25" hose barb (left) and the other for the 0.5" hose barb (right).
Tub 2: Sedimentation Box, with two (2) entrance and two (2) exit holes positioned at the top of the tub, both sides to accommodate 0.25" hose barb (left) and the other for the 0.5" hose barb (right).
Tub 3: Distribution Tank, with two (2) entrance and two (2) exit holes positioned at the top and bottom of the tub, respectively; both sides to accommodate one 0.25" hose barb (left) and the other for the 0.5" hose barb (right).
- Make copies of the Gravity-Fed System Design Worksheet, one per student.
- Display the Turbidity Chart (last slide in the presentation file) during the activity.
- Have the Gravity-Fed Water System Presentation (PowerPoint file) ready to show the class.
- Determine tub placement to allow for maximum flow; that is, Tub 1 on table, Tub 2 on chair next to table, Tub 3 on the floor.
With the Students
Day 1: Topic Introduction and Brainstorming (Assessment)
- Do the Pre-Activity Assessment with students (as described in the Assessment section). This connects with the beginning of the presentation, in which students learn a few important statistics regarding water poverty and some of the science behind waterborne disease.
- Show students the presentation; particularly direct their attention to the seriousness of water poverty, what causes waterborne illness, and how this problem truly needs a solution. Introduce the turbidity chart on the last slide.
- Provide students with the worksheets and briefly discuss the design activity. Provide an overview of what the activity entails: preliminary design, presentation to class, testing water system designs and changing. With the design, the goal is to have the highest flow (volume per time; that is, gallons per second), while having the greatest turbidity change.
Day 2: Preliminary Designing and Prototype Building
- Start class by reiterating the design activity (outlined in the worksheet).
- Divide the class into groups of six students each.
- Instruct them on how they can draw their preliminary designs and create a desired materials list (pipe sizes, tub elevations, pipe locations, etc.).
- Inform students that they can change the following parameters: tank elevations, tube diameters (sizes), and tube locations. Remind them how changing these parameters can affect the flow, where again, the goal of the activity is to have the water go from the top tank to the bottom tank the fastest, while changing the turbidity of the water as much as possible.
- Have students turn in their preliminary design and rationale (drawing and parts list) for approval.
- Leave enough time for the Activity Embedded Assessment (discussed in the Assessment section), which includes group presentations.
Day 3: Building and Testing the Prototype
- Demonstrate an example connecting the water system to tubs, and how water flows between tubs, by adding 1-gallon of water to the top tub (do not be partial to your design, just show the general design concept). Show exactly how you connect the tubes to the tub.
- Have stations set up for groups with boxes, tubes, tubs, dirt and cups. Attach hoses to classroom facets. If no faucet or convenient access to a faucet is available, fill up six (6) 5-gallon buckets and use those to fill Tub 1.
- Give groups time to build their gravity-fed water systems, guiding them through the steps or in helping to solve any challenges they encounter (see Figure 2).
- Once systems are fully put together, have teams test their designs without dirt to ensure water is flowing from tub to tub. This entails filling the top tub with about 1-gallon of water, and watching the water flow from top tub to bottom tub (Tubs 1 – 3) if working properly. Please take notice of any water leaking from improperly attached tubes—have a mop and bucket available for cleanup.
Figure 2. Students building their tub-tube assembly for their gravity-fed water systems. - Then place the full 2 cups of dirt in Tub 1; add 2 gallons of water; mix well while the system is beginning to flow. Have students refer to the turbidity chart to make note of how the water quality looks.
- Have students record the time it takes for water to transfer from Tub 1 into Tub 3, and note any complications within the tubs with regard to filling (that is, overflowing of one tub, one tub being emptied, etc.) on their worksheets.
- Once the water has stopped flowing, instruct students to declare the turbidity of water in Tub 3 (to ascertain how sedimentation affected turbidity), using Figure 3 for reference.
- Let students change their designs (see worksheet), and indicate their changes with their rationale behind it.
- Test the revised design, and make note of system performance, as indicated on the worksheet.
- Close with a 10-minute discussion on what students learned (see Post-Activity Assessment in the Assessment section). Be sure to focus on explicitly identifying which parameters caused the flow and sediment removal to be the most effective. Overall, ask students what they learned about the process of designing and building gravity-fed water systems.
Figure 3. A quick measurement guide for turbidity. Turbidity is measured by NTU (Nephelometric Turbidity Units). The higher the NTU, the cloudier the water.
Vocabulary/Definitions
bacteria: Microscopic single-celled organisms; may be beneficial or highly infectious to humans; in the case of waterborne illness, a harmful virus is E-coli.
diurnal water cycle: The amount of water available in a stream over the year.
flow: The amount of water flowing per unit time (volume/time) (cu. ft/s).
fluid density: The density of a fluid (water) which is mass (kg)/volume (cu.ft).
gravitational potential energy: The energy potential that exists due to relative vertical position.
kinetic energy: The energy of motion.
parasite: An organism (microscopic and macroscopic) that lives on a persons' nutrients; in the case of waterborne illness, common parasites are Guinea Worm, Cryptosporidiosis, and Giardiasis.
pore size: The size of the holes in a water filter; typically sized to let water pass through, while being too small to allow harmful organisms to pass as well.
pressure: A weight per area (lbs/sq.in).
roughness coefficient: Coefficients given to denote roughness (friction) in pipes.
sedimentation: The settling out of sediments by gravity in a water system.
virus: A very small organism that causes a disease to spread throughout the body; an example of a virus is Rotovirus (the stomach flu), or influenza.
water demand: The amount of water needed per person per day, summing by number of people in a community.
Assessment
Pre-Activity Assessment – Before Activity Presentations
What Is Water Poverty? Class Discussion: Ask the class: "What does water poverty mean to you?" Ask students to raise their hands to answer the question; select a few students to share their ideas. (Answer: water poverty is the condition of not having access to sufficient and/or clean water to meet one's basic needs.)
Then ask: "What do you think are some causes of water poverty?" Have students raise their hands to share their ideas. Write student ideas on the classroom board. (Possible answers are listed below.)
- Economic (Notice that water poverty does not exist in the U.S. "Why is that?")
- Political (Ask, "Why doesn't the government provide people with clean water?")
- Technical (Maybe the technology needed to provide clean water does not exist for a population. "Why not?")
- Environmental (Fact: In some areas of the world, no water may be available.)
Gravity-Fed Systems Brainstorming: Review brainstorming guidelines with students (no idea is silly, all ideas should be heard, withhold judgment, write down all ideas, etc.). In small groups, have students brainstorm what they think is meant by "gravity-fed water systems." Choose one person from each group to share his/her group's ideas.
Activity Embedded Assessment
Student-Generated Design Presentation: After groups have designed their water systems, direct them to prepare very short (verbal) presentations to discuss their general design ideas with the class. Ask each group the following questions to gauge their understanding of gravity-fed systems:
- How does your design ensure maximum flow, flow equalization in tanks, and maximum sediment removal?
- What are possible complications that might arise?
- How might you change the design if these complications take place?
Post-Activity Assessment
Project Reflections: After testing their systems, put the following questions on the classroom board and give students time to write answers based on their wrap-up thoughts from the activity.
- What design considerations seemed to work best— not just in your group but across all groups?
- What problems did it seem like all groups had, including yours?
- What do you think is the hardest part about gravity-fed water system design? <
Safety Issues
- Have students wear eye protection throughout the activity. Even though the lab only deals with water, expect spills, spraying and splashing of the dirty water. Remind students that wearing eye protection is a necessary habit when dealing with liquids in any lab setting.
Troubleshooting Tips
- Experiment with the water system building in advance to ensure the materials all work together and that no leaks occur and the tubes fit.
- To avoid delays, have ready a model prototype to show at the beginning of Day 2.
- During student testing, quickly take notice of water leaking from incorrectly attached tubes; have a mop and bucket available for cleanup.
Activity Scaling
- For lower grades, have students spend less time with the design process and presentations, and more time building and testing their prototypes.
- For upper grades, have students take more time with the design process, focusing more on the concepts articulated in the presentations and gathered from intuition and theories presented by their classmates in the pre-build discussion on Day 2.
- For upper grades, incorporate a final design stage in which each student draws his/her final designs based on what s/he learned from the testing and presentations. Have them turn in their designs for grading or participation marks.
Subscribe
Get the inside scoop on all things TeachEngineering such as new site features, curriculum updates, video releases, and more by signing up for our newsletter!More Curriculum Like This

Students learn about water quality testing and basic water treatment processes and technology options. Biological, physical and chemical treatment processes are addressed, as well as physical and biological water quality testing, including testing for bacteria such as E. coli.

Students learn about the various methods developed by environmental engineers for treating drinking water in the United States.

Students experience firsthand one of the most common water treatment types in the industry today, flocculants. They learn how the amount of suspended solids in water is measured using the basic properties of matter and light. In addition, they learn about the types of solids that can be found in wat...
Copyright
© 2013 by Regents of the University of ColoradoContributors
Jeff Walters, Malinda Schaefer Zarske, Janet YowellSupporting Program
Integrated Teaching and Learning Program, College of Engineering, University of Colorado BoulderAcknowledgements
This digital library content was developed by the Integrated Teaching and Learning Program under National Science Foundation GK-12 grant no. DGE 0338326. However, these contents do not necessarily represent the policies of the NSF, and you should not assume endorsement by the federal government.
Last modified: April 4, 2019
User Comments & Tips