Quick Look
Grade Level: 11 (8-11)
Time Required: 30 minutes
(one-half class period)
Expendable Cost/Group: US $0.50 Activity requires the use of non-expendable materials (at least three different particle sizes of quantum dot solutions, which may last up to two years, plus black light and lamp); see Materials List for options and details.
Group Size: 3
Activity Dependency: None
Subject Areas: Chemistry, Physics
NGSS Performance Expectations:
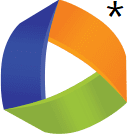
HS-PS2-6 |
Summary
Students are introduced to the physical concept of the colors of rainbows as light energy in the form of waves with distinct wavelengths, but in a different manner than traditional kaleidoscopes. Looking at different quantum dot solutions, they make observations and measurements, and graph their data. They come to understand how nanoparticles interact with absorbing photons to produce colors. They learn the dependence of particle size and color wavelength and learn about real-world applications for using these colorful liquids.Engineering Connection
Quantum dots are being used in many real-world applications such as cancer treatments and solar energy generation. Cancer treatments take advantage of the UV absorption ability of different-sized quantum dots to release specific wavelengths of light for detection and monitoring purposes. Traditional polycrystalline solar cells are expensive and limited in efficiency; quantum dots allow engineers to tailor properties to absorb all energy from the Sun (UV-IR) and produce electrical power. The advantages of using quantum dots included enhanced surface area for absorption and particle size-absorption interactions.
Learning Objectives
After this activity, students should be able to:
- Describe colors in terms of light energy and wavelength.
- Describe how quantum dots work.
- Describe the interplay between quantum dot particle size and color.
- Explain some practical applications for quantum dots.
Educational Standards
Each TeachEngineering lesson or activity is correlated to one or more K-12 science,
technology, engineering or math (STEM) educational standards.
All 100,000+ K-12 STEM standards covered in TeachEngineering are collected, maintained and packaged by the Achievement Standards Network (ASN),
a project of D2L (www.achievementstandards.org).
In the ASN, standards are hierarchically structured: first by source; e.g., by state; within source by type; e.g., science or mathematics;
within type by subtype, then by grade, etc.
Each TeachEngineering lesson or activity is correlated to one or more K-12 science, technology, engineering or math (STEM) educational standards.
All 100,000+ K-12 STEM standards covered in TeachEngineering are collected, maintained and packaged by the Achievement Standards Network (ASN), a project of D2L (www.achievementstandards.org).
In the ASN, standards are hierarchically structured: first by source; e.g., by state; within source by type; e.g., science or mathematics; within type by subtype, then by grade, etc.
NGSS: Next Generation Science Standards - Science
NGSS Performance Expectation | ||
---|---|---|
HS-PS2-6. Communicate scientific and technical information about why the molecular-level structure is important in the functioning of designed materials. (Grades 9 - 12) Do you agree with this alignment? |
||
Click to view other curriculum aligned to this Performance Expectation | ||
This activity focuses on the following Three Dimensional Learning aspects of NGSS: | ||
Science & Engineering Practices | Disciplinary Core Ideas | Crosscutting Concepts |
Communicate scientific and technical information (e.g. about the process of development and the design and performance of a proposed process or system) in multiple formats (including orally, graphically, textually, and mathematically). Alignment agreement: | Attraction and repulsion between electric charges at the atomic scale explain the structure, properties, and transformations of matter, as well as the contact forces between material objects. Alignment agreement: | Investigating or designing new systems or structures requires a detailed examination of the properties of different materials, the structures of different components, and connections of components to reveal its function and/or solve a problem. Alignment agreement: |
Common Core State Standards - Math
-
Construct and interpret scatter plots for bivariate measurement data to investigate patterns of association between two quantities. Describe patterns such as clustering, outliers, positive or negative association, linear association, and nonlinear association.
(Grade
8)
More Details
Do you agree with this alignment?
-
Solve simple rational and radical equations in one variable, and give examples showing how extraneous solutions may arise.
(Grades
9 -
12)
More Details
Do you agree with this alignment?
-
Represent data on two quantitative variables on a scatter plot, and describe how the variables are related.
(Grades
9 -
12)
More Details
Do you agree with this alignment?
International Technology and Engineering Educators Association - Technology
-
Conduct research to inform intentional inventions and innovations that address specific needs and wants.
(Grades
9 -
12)
More Details
Do you agree with this alignment?
State Standards
Texas - Science
-
investigate and analyze characteristics of waves, including velocity, frequency, amplitude, and wavelength, and calculate using the relationship between wavespeed, frequency, and wavelength;
(Grades
9 -
12)
More Details
Do you agree with this alignment?
-
understand the electromagnetic spectrum and the mathematical relationships between energy, frequency, and wavelength of light;
(Grades
10 -
12)
More Details
Do you agree with this alignment?
Materials List
Each group needs:
- cardboard shoe box
- black light bulb (such as Smart Electric 60W A19 black light bulb, model #110 for $5.02 at The Home Depot)
- desk lamp compatible with A19 type bulb (suitable lamps may be available in school labs)
- black construction paper, 4 sheets
- test tube holder (or similar fixture) for holding quantum dot vials
- stopwatch
- Quantum Dots and Colors Worksheet, one per student
To share with the entire class:
- quantum dots of various particle sizes (obtain a minimum of three different particle sizes; see suggested sources listed below, such as LumidotTM quantum dot kits, which include various size ranges for demonstrations)
- 2 packages of 50, 9 x12-inch black construction paper ($1.49/pack, available at Office Depot)
- masking tape and marker, to label vials with particle size of solution
- tape (or glue)
- scissors
Three source options for quantum dot solutions:
- CDSe 5 ml solutions individually purchased for $194 per 5 ml solution, such as part #662429 at SigmaAldrich https://www.sigmaaldrich.com/
- LumidotTM CDSe-6 quantum dot nanoparticles kit for $687, such as #662593-1KT at SigmaAldrich https://www.sigmaaldrich.com/
- Contact a local university biochemistry or chemistry program to request sample quantum dot solutions for classroom use; ask for a minimum of 5-10 ml per solution per group
Worksheets and Attachments
Visit [www.teachengineering.org/activities/view/uoh_nano_lesson02_activity3] to print or download.Pre-Req Knowledge
Students must be able to perform simple algebra. It is helpful if students have been introduced to basic physics involving wavelengths and frequencies.
Introduction/Motivation
(Present the following information to the class, or else have students read it themselves as they conduct the activity experiment, since it is provided on the attached worksheet.)
Quantum dots are semiconducting nanoparticles that are able to confine electrons in small, discrete spaces. Also known as "zero-dimensional electronic structures," quantum dots are unique in that their semiconductor energy levels can be tailored by simply altering size, shape and charge potential. These energy levels result in distinct color identifications for different-sized quantum dots.
Semiconductors rely on electron excitation from the ground state into some excited energy level. The space between the excited and ground states is a characteristic energy band gap. When an electron is excited (ejected from ground state), a corresponding hole (positive charge) is left in the wake. The separation between the excited electrons (negative charge) sets up a potential difference. When this occurs and a circuit is completed, electron flow begins in the conductive band of the semiconductor. To re-establish the ground state, electrons and holes recombine, emitting photons over a wide and continuous wavelength range. However, this behavior in a bulk semiconductor primarily occurs over a range of energy levels and is limited by dimensions of the absorbing surface and range of incident photon wavelength.
Quantum dots offer a highly efficient process that mimics that of a bulk semiconductor but is quantized because of the length scales involved. "Quantum confinement" allows for quantum dots to be tailored to specific incident energy levels based on particle size. Additionally, nanoparticles offer superior surface area increases that enhance absorption properties per unit volume and/or conductive properties. Material scientists have found by varying quantum dot size, energy band gap size may increase or decrease. Larger size quantum dots create a decrease in energy band gap and emit large wavelength photons (red-shift). Small quantum dot sizes have an increase in energy band gap and emit short wavelength light (blue shift). This effect is demonstrated by quantum dot solutions of different particle sizes emitting different colors when exposed to a UV light source. Surface area effects enhance the efficiency of energy transfer properties, hence, quantum dots are known to have "high quantum yield." This also pertains to quantum dots emitting light long after exposure to a UV energy source.
Because of the unique quantum confinement effect, quantum dots are suitable for variety of applications, including medical and energy applications. Tumor or cancer detection is a primary application for quantum dots. Scientists attach different antibodies to specific proteins and release them. When quantum dots have permeated cell walls, scientists tag their locations through fluorescence imaging. Additionally, because of the high quantum yield, quantum dots remain fluorescent over time, making them useful for imaging purposes. Solar energy is another targeted application for quantum dots. Specifically, quantum dots are of interest for their exceptional and tailored light optical properties. Additionally, a robust solar cell may be easily manufactured consisting of optical layers of differing quantum dot sizes to absorb specific incident photons. This allows solar cells to utilize all wavelengths of light provided by the Sun, boosting solar cell efficiencies
These are only a few of numerous applications. However, to really understand quantum dots, you must observe them first-hand. This activity provides the basis for understanding how these tiny optical beacons have the potential to become important to our way of life in the near future. As scientists and engineers, it is important to record all observations and draw conclusions from those observations. So observe carefully and have fun!
Procedure
Before the Activity
- Collect materials and make copies of the Quantum Dots and Colors Worksheet, one per student.
- Collect enough shoe boxes so you have one per group; ask students to bring from home, if available.
- Prepare each shoe box into a black box with a viewing slot and cord hole:
- On the long side of each box, cut a 1.5-in x 4.5-in slit.
- On the adjacent (small) box face near the lower corner furthest away from long-side slit, cut a 1-in x 1-in hole (for the light cord to pass through).
- Use tape or glue to cover the entire box interior with black construction paper, making sure to keep the holes free from obstructions.
- Assemble a black light into the box with the cord extending out the small hole.
- Place a test tube holder (or similar fixture) inside the box to hold the quantum dot vials.
- Use tape and a marker to label each vial of quantum dot solution with its particle size.
With the Students—Overall Procedure
- Divide the class into groups of three or four students each. Hand out the viewing boxes, black lights, stopwatches, test tube holders and worksheets.
- Instruct students to review the materials list on the worksheet to make sure they have what they need.
- Pass out one vial of quantum dots solution to each group.
- Have students read the worksheet, follow its instructions and answer its questions.
- Have students turn in their worksheets for grading.
- Conclude with a class discussion to compare results and conclusions.
Student Procedure (also on the worksheet)
- Record the quantum dot particle size on the worksheet.
- Place the quantum dot solution into the holder located inside the box.
- Turn on the black light and close the box lid.
- Looking through the long view port, observe the quantum dot solution when exposed to black light. Record its color and associated wavelength.
- Turn the black light off and observe the solution for 1 minute. Record your observations.
- After all observations have been recorded, remove the quantum dot solution. Trade your vial with another group so you get a different quantum dot solution.
- Repeat steps 1-5 until you have observed and collected data for all quantum dot solutions circulating.
- Make sure to fill in all worksheet blanks with the appropriate information.
- Complete all worksheet questions and turn in when finished.
- Return all quantum dot solutions to the instructor and clean up activity station.
Vocabulary/Definitions
band gap: A band gap is the energy required to excite an electron into the conductive band. It is the energy gap between conductive and valence bands. The size is characteristic of the material. For conductive materials, the band gap is non-existent and both conductive and valence bands overlap. In semiconductor materials, both valence and conductive bands are separated.
conductive band: The semiconductor energy band to which electrons are transferred when excited from the valence band.
electromagnetic radiation: A means of energy travel through a vacuum. Has characteristic wavelengths and frequencies.
fluorescence : Emission of light by a substance that absorbs light by electromagnetic radiation of a different wavelength.
holes: A positively charged space that is created when an electron is excited and moving. Holes can move freely about a crystal. Typically, they are separated and form in p-type semiconductors.
quantum confinement: An effect created by decreasing particles to the same magnitude as the particle wave function. Cause for change in electrical and optical properties.
quantum dots: Semiconducting nanoparticles that are able to confine electrons in small, discrete spaces.
quantum yield: The number of times an event occurs per photon absorbed by the system.
valence band: The semiconductor energy band to which holes are transferred when electrons are excited and transferred to the conductive band.
Assessment
Activity Embedded Assessment
Worksheet: The attached Quantum Dots and Colors Worksheet is designed to guide students in recording observations, analyzing their observations and drawing conclusions based on observations. Through this worksheet, they explore more general physics concepts on light energy, wavelength, frequency and quantum confinement while using energy and kinetic energy principles. Students need to read questions thoroughly to identify pertinent information to solve each problem. Expect students to finish the worksheet in class.
Post-Activity Assessment
Worksheet: Have students turn in their completed worksheets for grading. Review their answers to gauge their mastery of the concepts.
Closing Class Discussion: Lead a post-activity discussion to compare results and conclusions, including answers to the worksheet questions.
Safety Issues
- All supplies used in this activity are harmless if maintained in storage vials. Handle quantum dot solution vials with care and abide by laboratory safety and handling procedures as necessary.
Subscribe
Get the inside scoop on all things TeachEngineering such as new site features, curriculum updates, video releases, and more by signing up for our newsletter!More Curriculum Like This

Through three teacher-led demonstrations, students are shown samplers of real-world nanotechnology applications involving ferrofluids, quantum dots and gold nanoparticles. This nanomaterials engineering lesson introduces practical applications for nanotechnology and some scientific principles relate...

Students learn about the biomedical use of nanoparticles in the detection and treatment of cancer, including the use of quantum dots and lasers that heat-activate nanoparticles. They also learn about electrophoresis—a laboratory procedure that uses an electric field to move tiny particles through a ...

Student teams conduct an experiment that uses gold nanoparticles as sensors of chemical agents to determine which of four sports drinks has the most electrolytes. Using some basic chemistry and physics principles, students develop a conceptual understanding of how gold nanoparticles function.
References
Lindsay, Stuart .M. Introduction to Nanoscience. New York, NY: Oxford University Press, Inc., 2010, pp. 318-335.
Sanderson, Katharine. "Quantum dots go large: A small industry could be on the verge of a boom," Special Report, Nature. Macmillan Publishers Limited. Vol. 459, No. 11, pp. 760-761; June 2009. Accessed September 20, 2012 http://unanotech.com/wp-content/uploads/2011/06/Nature_Quantum_dots_go_large.pdf
Williams, Linda and Wade Adams. Nanotechnology Demystified. New York, NY: McGraw-Hill, 2007, pp. 146-149.
Copyright
© 2013 by Regents of the University of Colorado; original © 2011 University of HoustonContributors
Marc BirdSupporting Program
National Science Foundation GK-12 and Research Experience for Teachers (RET) Programs, University of HoustonAcknowledgements
This curriculum was created by the University of Houston's College of Engineering with the support of National Science Foundation GK-12 grant no. DGE 0840889. However, these contents do not necessarily represent the policies of the National Science Foundation, and you should not assume endorsement by the federal government.
Last modified: January 10, 2019
User Comments & Tips