Quick Look
Grade Level: 11 (10-12)
Time Required: 1 hours 45 minutes
(two 50-minute class periods)
Expendable Cost/Group: US $15.00
Group Size: 3
Activity Dependency: None
Subject Areas: Biology, Data Analysis and Probability, Life Science, Measurement
NGSS Performance Expectations:
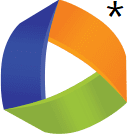
HS-LS1-1 |
Summary
This engineering design activity simulates building a model to visualize and measure DNA damage within the nucleus as the cell migrates through the extracellular matrix (ECM). As students learn more about the structural composition of the ECM and the nucleus, they come to understand that both the ECM and the nucleus vary in stiffness and elasticity. Mechanobiologists have recently discovered that this feature plays an influential role in regulating numerous cell functions, as cells have the ability to “sense” their mechanical environment. The stiffness of the ECM is particularly important in the spread of cancer throughout the body. In this activity, students model the movement of a cell through a stiff matrix and measure the impact on the nucleus as DNA damage caused by the stress of this movement in relation to the amount of nuclear lamins, the major architectural proteins of the animal cell nucleus, present.Engineering Connection
Mechanobiology is an emerging field of science that mixes biology, engineering, and physics. Mechanobiologists study how cells, tissues, and organs are constantly exposed to internal and external forces (or biomechanical cues) which guide and impact structure and function of those cells. Understanding what these biomechanical cues are and how cells respond has important implications for diseases such as cancer. Studying these forces provides insight on how cancers progress as well as on potential new treatments and therapies.
Learning Objectives
After this activity, students should be able to:
- Explain how there are tissues of varying stiffness in the human body.
- Describe how cells can become strained as they migrate through the extracellular matrix.
- Build a model that demonstrates how cell migration can lead to DNA damage due to the structural make-up of the nucleus and the surrounding forces acting upon the cell.
- Explain the connection between cell stress, DNA damage, and metastasis.
Educational Standards
Each TeachEngineering lesson or activity is correlated to one or more K-12 science,
technology, engineering or math (STEM) educational standards.
All 100,000+ K-12 STEM standards covered in TeachEngineering are collected, maintained and packaged by the Achievement Standards Network (ASN),
a project of D2L (www.achievementstandards.org).
In the ASN, standards are hierarchically structured: first by source; e.g., by state; within source by type; e.g., science or mathematics;
within type by subtype, then by grade, etc.
Each TeachEngineering lesson or activity is correlated to one or more K-12 science, technology, engineering or math (STEM) educational standards.
All 100,000+ K-12 STEM standards covered in TeachEngineering are collected, maintained and packaged by the Achievement Standards Network (ASN), a project of D2L (www.achievementstandards.org).
In the ASN, standards are hierarchically structured: first by source; e.g., by state; within source by type; e.g., science or mathematics; within type by subtype, then by grade, etc.
NGSS: Next Generation Science Standards - Science
-
Use a model to predict the relationships between systems or between components of a system.
(Grades 9 - 12)
More Details
Do you agree with this alignment?
-
Use mathematical and/or computational representations of phenomena or design solutions to support explanations.
(Grades 9 - 12)
More Details
Do you agree with this alignment?
NGSS Performance Expectation | ||
---|---|---|
HS-LS1-1. Construct an explanation based on evidence for how the structure of DNA determines the structure of proteins which carry out the essential functions of life through systems of specialized cells. (Grades 9 - 12) Do you agree with this alignment? |
||
Click to view other curriculum aligned to this Performance Expectation | ||
This activity focuses on the following Three Dimensional Learning aspects of NGSS: | ||
Science & Engineering Practices | Disciplinary Core Ideas | Crosscutting Concepts |
Construct an explanation based on valid and reliable evidence obtained from a variety of sources (including students' own investigations, models, theories, simulations, peer review) and the assumption that theories and laws that describe the natural world operate today as they did in the past and will continue to do so in the future. Alignment agreement: | Systems of specialized cells within organisms help them perform the essential functions of life. Alignment agreement: All cells contain genetic information in the form of DNA molecules. Genes are regions in the DNA that contain the instructions that code for the formation of proteins, which carry out most of the work of cells.Alignment agreement: | Investigating or designing new systems or structures requires a detailed examination of the properties of different materials, the structures of different components, and connections of components to reveal its function and/or solve a problem. Alignment agreement: |
Common Core State Standards - Math
-
Summarize, represent, and interpret data on two categorical and quantitative variables
(Grades
9 -
12)
More Details
Do you agree with this alignment?
-
Represent data on two quantitative variables on a scatter plot, and describe how the variables are related.
(Grades
9 -
12)
More Details
Do you agree with this alignment?
Materials List
Each group needs:
- three 30 cm balloons
- permanent marker
- empty water bottle
- 500-700 hydrogel “Sooper beads” (available online)
- 3 small beakers (large enough to hold 150-200 beads)
- three plastic shower curtain rings
- handful of rotini pasta (about 20-25 pieces)
- one pair of scissors
- large tray or box for assessing hydrogel damage
Worksheets and Attachments
Visit [www.teachengineering.org/activities/view/uop-2575-modeling-nuclear-damage-cell-movement] to print or download.Pre-Req Knowledge
Students should understand the relationship of cells and proteins. Students should also understand basic cell structure and the function of the cytoskeleton.
Introduction/Motivation
How many of you have heard of the term mechanobiology? Did you know that there are researchers and medical doctors who combine their knowledge of physics, engineering and biology to study how the forces acting on cells influence how cells operate? This is what mechanobiologists do.
What can we learn from studying the forces acting on cells and changes in the mechanical properties of cells and tissues? Understanding these effects allows mechanobiologists to engineer new tissues and organs, repair and regenerate damaged tissue, integrate artificial implants with native tissue in reconstructive surgery, and provide therapy for inflammatory diseases.
Researchers have found that the extracellular matrix (ECM), the network of collagen, other proteins, and carbohydrates outside of a cell, plays an important role in the mechanical environment of cells and can impact the design of new tissues and treatment of diseases such as cancer. Cells are connected through linker proteins called integrins to their ECMs at cell–ECM adhesion sites, the points where cells physically attach to their environment. These adhesion sites are linked through the cell membrane to the cytoskeleton (the internal skeleton of the cell, made of fibers of a protein called actin, among other proteins), and this enables the cell to respond to external stimuli in a coordinated manner. Biomechanical cues from the ECM through cell-adhesion proteins like integrins are fundamental for defining the invasive potential of cancer cells, and many of these proteins have been proposed as potential targets for inhibiting cancer cell invasion and thus, metastasis.
Note that the ECM can exist in varying degrees of stiffness, from soft (as in brain) to hard (as in bone); this is primarily dependent on the concentrations of collagen, a rigid protein, and elastin (you guessed it – an elastic protein). The accumulation of collagen fibers results in a stiffer ECM and is considered a typical characteristic of cancerous tumors. Stiffening is not only associated with tumor cells displacing the host tissue and growing in size but also contributes to cell–ECM interactions and can promote tumor cell invasion to surrounding tissues.
In this activity, we will explore the direct damage to DNA that is caused when cells migrate through a stiff ECM – in other words, we’ll learn one potential mechanism for how a stiff environment outside a cell can lead to potentially permanent damage to DNA inside of the cell. Surrounding the nucleus is a structure called the nuclear lamina, which is a dense fibrous network found in most animal cells made of filaments and proteins that surrounds the nucleus and gives the nucleus its shape. This network not only provides mechanical support, but is also important in regulating cellular events such as DNA replication and cell division. Since there is a relationship between the lamina and DNA function, damage to the lamina can contribute to DNA malfunction or incorrect DNA replication, which can lead to cancers.
Our experiment today will simulate blebbing. Blebbing occurs when a cell deforms in shape when it passes through a small space such as the spaces of the extracellular matrix. Imagine what happens when you squeeze a stress ball… it loses its circular shape. In some cases where cells are squeezed, lamins also feel the stress and the nucleus loses its circular shape, leading to DNA damage. The amount of lamins in a cell will change the stiffness of the nucleus and therefore impact damage to the DNA when the cell is migrating. By modeling varying amounts of lamins in a nucleus in this experiment, we will simulate the change in stress the nucleus undergoes during blebbing and visualize the damaging effects on the DNA contained within the cell’s nucleus.
Procedure
Background
(See the Extracellular Matrix and Molecular Biomechanics PowerPoint).
In biology, the extracellular matrix (ECM) is a three-dimensional network of extracellular macromolecules, such as collagen, other proteins and enzymes, and carbohydrates, that provides structural and biochemical support to surrounding cells. Because multicellularity evolved independently in different organisms, the composition of ECM varies between multicellular structures; however, cell adhesion, cell-to-cell communication, and differentiation are common functions of the ECM.
Cells can sense the mechanical properties of their environment by applying forces and sensing the resulting backlash. This helps regulate many important cellular processes including cellular contraction, cell migration, cell proliferation, differentiation, and cell death (apoptosis).
Metastasis is a multistep process in which tumor extracellular matrix (ECM) and cancer cell cytoskeleton interactions are important components. The ECM is connected, through integrins, to the cell at cell–ECM adhesion sites and to the actin cytoskeleton and various signaling pathways enabling the cell to respond to external stimuli in a coordinated manner. Cues from cell-adhesion proteins are fundamental for defining the invasive potential of cancer cells, and many of these proteins have been proposed as potent targets for inhibiting cancer cell growth and metastasis. In addition, the accumulation of collagen in the ECM is common within the tumor microenvironment leading to an intense fibrotic response, known as desmoplasia, and tumor stiffening. Stiffening is not only required for the tumor to be able to displace the host tissue and grow but also contributes to cell–ECM interactions and can promote cancer cell invasion to surrounding tissues.
In this lab, you are going to model the movement of different cells, whose nuclei are composed of varying amounts of lamins, through a matrix and measure the DNA damage caused by the forces on the cell.
To the teacher: The components of this model are as follows: Balloons will represent the cell nucleus. Students will add pasta pieces (representing nuclear lamins) and hydrogel beads (representing chromatin or DNA). Students will push three different ‘nuclei’ with varying lamin concentrations through a plastic shower curtain ring (representing openings in the ECM). DNA damage will be simulated by ruptured or torn hydrogels beads.
Before the Activity
- Gather materials and make copies of the Functions of the ECM Pre-Activity and Post-Activity Assessment.
- Place hydrogel beads into the beaker and cover with water. Allow beads to sit overnight to rehydrate.
With the Students
Day 1
- Review the Extracellular Matrix and Molecular Biomechanics PowerPoint, Background material, and Introduction.
- Have students the complete the Functions of the ECM Pre-Activity.
Day 2
- Divide class into groups of 3 students each - Each group will create three model nuclei.
- Get three 30 cm balloons. Label #1 as “0”; #2 as “5”; and #3 as “10”.
- Add 5 rotini pasta pieces to balloon #2 and 10 rotini to balloon #3. Partially inflate each balloon and loosely twist the top to hold air in—do NOT tie off!
- Carefully count out 150-200 hydrogels each into each of three small beakers (make sure each beaker has the same amount).
- Pour one set of the hydrogels into an empty water bottle.
- Place the opening of balloon #1 on top of the water bottle and transfer the hydrogels into the balloon.
- Release any extra air from the balloon and tie off the opening.
- Repeat steps 5 through 7 for balloons #2 and #3.
- Write your predictions about what will happen to each balloon and/or its contents on a data table (e.g., will there be damage, which would have more damage, and why?)
- Take each balloon, one at a time, and push them through the shower ring.
- Once completed, cut open each balloon (be careful to not to damage the hydrogels inside), and place the contents into a large tray.
- Sort and count the hydrogels into two groups: DAMAGED and NOT DAMAGED. Only count whole hydrogels as ‘not damaged’.
- Record your data on a shared class datasheet and construct a graph comparing average DNA damage (including error bars) across different lamin protein levels.
- Have students complete the and Post-Activity Assessment.
Vocabulary/Definitions
blebbing: Bulging that occurs when a cell deforms in shape as it passes through a small space such as the spaces of the extracellular matrix.
cell adhesion: The process by which cells form contacts with each other or with their substrates through specialized protein complexes.
collagen: A key protein of the extracellular matrix; the primary structural component of connective tissues, such as skin and cartilage.
elastin: A key protein of the extracellular matrix. It is highly elastic and present in connective tissue allowing many tissues in the body to resume their shape after stretching or contracting.
extracellular matrix: A three-dimensional network of extracellular macromolecules, such as collagen, enzymes, and glycoproteins, that provide structural and biochemical support between cells.
lamins: Major architectural proteins of the animal cell nucleus. Lamins line the inside of the nuclear membrane, where they provide a platform for the binding of proteins and chromatin and confer mechanical stability. They have been implicated in a wide range of nuclear functions, including higher-order genome organization, chromatin regulation, transcription, DNA replication and DNA repair.
mechanobiology: An emerging field of science at the interface of biology, engineering, and physics that focuses on how physical forces impact the mechanical properties of cells and tissues and contribute to development, cell differentiation, physiology, and disease.
mechanosensing: Responsivity to mechanical stimuli, especially at the cellular level or below.
metastasis: The development of secondary cancer growth at a distance from the main site of cancer.
nuclear blebs: Protrusions from the nuclear membrane.
Assessment
Pre-Activity Assessment
Pre-Activity: Have students complete the Functions of the ECM Pre-Activity.
Activity Embedded (Formative) Assessment
Questions: Ask students the following questions as they complete the activity.
- Predict what will happen within each model cell as it migrates.
- Construct a graph comparing DNA damage with different lamin protein levels.
Post-Activity (Summative) Assessment
Post-Assessment: Have students complete the Post-Activity Assessment.
Activity Extensions
Have students complete the Extension Activity: Learning More about Metastasis and ECM Rigidity.
Additional Multimedia Support
Video on the Extracellular Matrix from Khan Academy: https://www.khanacademy.org/science/biology/structure-of-a-cell/cytoskeleton-junctions-and-extracellular-structures/v/extracellular-matrix
Article in Nature Communications, “Cancer cells with trapped nuclei cut their way through the extracellular matrix,” by Emmanuel Dornier & Jim C. Norman: https://www.nature.com/articles/s41467-018-06351-6
Subscribe
Get the inside scoop on all things TeachEngineering such as new site features, curriculum updates, video releases, and more by signing up for our newsletter!References
Cox, Thomas & Erler, Janine. “Remodeling and homeostasis of the extracellular matrix: implications for fibrotic diseases and cancer.” Disease Models & Mechanisms. (2011) Vol. 4,pp. 165-78. 10.1242/dmm.004077
Dornier, Emmanuel, & Norman, Jim C. “Cancer cells with trapped nuclei cut their way through the extracellular matrix.” Nature Communications. (2018) Vol. 9, p. 1-3 . https://doi.org/10.1038/s41467-018-06351-6
Irianto, Jerome, Xia, Yuntao, Pfeifer, Charlotte R., Athirasala, Avathamsa, Ji, Jiazheng, Alvey, Cory, Tewari, Manu, Bennett, Rachel R., Harding, Shane M., Liu, Andrea J., Greenberg, Roger A., & Discher, Dennis E. “DNA damage follows repair factor depletion and portends genome variation in cancer cells after pore migration.” Current Biology (2017), Vol. 27, pp. 210-23. https://doi.org/10.1016/j.cub.2016.11.049
Perelman School of Medicine, University of Pennsylvania. “Tension on gut muscles induces cell invasion in zebrafish intestine, mimicking cancer metastasis.” Science Daily. Sep 2012, Vol 7. https://www.eurekalert.org/pub_releases/2012-09/uops-tog090712.php
Copyright
© 2021 by Regents of the University of Colorado; original © 2019 University of PennsylvaniaContributors
Elizabeth Hale; Manasvita Vashisth; Amanda Cottone; James McGonigle; Rebecca WellsSupporting Program
Science and Technology Center for Engineering Mechano-Biology RET, University of PennsylvaniaAcknowledgements
This material was developed based upon work supported by the National Science Foundation under grant no. 1548571—Science and Technology Center for Engineering Mechano-Biology, from the Civil, Mechanical and Manufacturing Innovation (CMMI) at the University of Pennsylvania. Any opinions, findings, and conclusions or recommendations expressed in this material are those of the authors and do not necessarily reflect the views of the National Science Foundation.
Last modified: May 26, 2021
User Comments & Tips