Quick Look
Grade Level: 11 (10-12)
Time Required: 1 hours 45 minutes
Two 50-minute periods
Lesson Dependency: None
Subject Areas: Physics, Science and Technology
NGSS Performance Expectations:
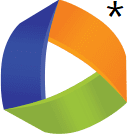
HS-PS2-1 |
Summary
Students apply their mathematics and team building skills to explore the concept of rocketry. They learn about design issues faced by aerospace engineers when trying to launch rocketships or satellites in order to land them safely—in the ocean, for example. Students learn the value of designing within constraints while brainstorming a rocketry system using provided materials and a specified project budget. Throughout the design process, teamwork is emphasized since the most successful launches occur when groups work effectively to generate creative ideas and solutions to the rocket challenge.Engineering Connection
Engineers study lift, thrust, gravity and drag so that rockets and shuttles can launch into space and planes can fly. Engineers continually work on planes to improve their design and reliability and to generally make air travel safer. The space shuttle continues exploring space, and newly engineered rovers, deep space telescopes, and space probes unveil new discoveries as we enter the next century. Rockets make this vital space exploration possible. Also, rockets deliver satellites—for example those that enable cell phones to find signals and make/receive phone calls—and are expected to continue to be an integral part of our expanding communications systems. These are but a few glimpses into the importance of understanding the physics and engineering behind flight so that rockets can continue to expand our horizons.
Learning Objectives
After this lesson, students should be able to:
- Relate rocket motion to Newton's laws of motion.
- Draw free-body diagrams for s rocket through various stages of flight.
- Calculate the time to descend in free fall.
- Explain the parameters that go into rocket design.
- Work independently and function as a team.
Educational Standards
Each TeachEngineering lesson or activity is correlated to one or more K-12 science,
technology, engineering or math (STEM) educational standards.
All 100,000+ K-12 STEM standards covered in TeachEngineering are collected, maintained and packaged by the Achievement Standards Network (ASN),
a project of D2L (www.achievementstandards.org).
In the ASN, standards are hierarchically structured: first by source; e.g., by state; within source by type; e.g., science or mathematics;
within type by subtype, then by grade, etc.
Each TeachEngineering lesson or activity is correlated to one or more K-12 science, technology, engineering or math (STEM) educational standards.
All 100,000+ K-12 STEM standards covered in TeachEngineering are collected, maintained and packaged by the Achievement Standards Network (ASN), a project of D2L (www.achievementstandards.org).
In the ASN, standards are hierarchically structured: first by source; e.g., by state; within source by type; e.g., science or mathematics; within type by subtype, then by grade, etc.
NGSS: Next Generation Science Standards - Science
NGSS Performance Expectation | ||
---|---|---|
HS-PS2-1. Analyze data to support the claim that Newton's second law of motion describes the mathematical relationship among the net force on a macroscopic object, its mass, and its acceleration. (Grades 9 - 12) Do you agree with this alignment? |
||
Click to view other curriculum aligned to this Performance Expectation | ||
This lesson focuses on the following Three Dimensional Learning aspects of NGSS: | ||
Science & Engineering Practices | Disciplinary Core Ideas | Crosscutting Concepts |
Analyze data using tools, technologies, and/or models (e.g., computational, mathematical) in order to make valid and reliable scientific claims or determine an optimal design solution. Alignment agreement: Theories and laws provide explanations in science.Alignment agreement: Laws are statements or descriptions of the relationships among observable phenomena.Alignment agreement: | Newton's second law accurately predicts changes in the motion of macroscopic objects. Alignment agreement: Attraction and repulsion between electric charges at the atomic scale explain the structure, properties, and transformations of matter, as well as the contact forces between material objects.Alignment agreement: | Empirical evidence is required to differentiate between cause and correlation and make claims about specific causes and effects. Alignment agreement: |
Common Core State Standards - Math
-
Attend to precision.
(Grades
K -
12)
More Details
Do you agree with this alignment?
-
Model with mathematics.
(Grades
K -
12)
More Details
Do you agree with this alignment?
-
Solve linear equations and inequalities in one variable, including equations with coefficients represented by letters.
(Grades
9 -
12)
More Details
Do you agree with this alignment?
-
Summarize, represent, and interpret data on a single count or measurement variable
(Grades
9 -
12)
More Details
Do you agree with this alignment?
-
Use units as a way to understand problems and to guide the solution of multi-step problems; choose and interpret units consistently in formulas; choose and interpret the scale and the origin in graphs and data displays.
(Grades
9 -
12)
More Details
Do you agree with this alignment?
-
Reason quantitatively and use units to solve problems.
(Grades
9 -
12)
More Details
Do you agree with this alignment?
-
Rearrange formulas to highlight a quantity of interest, using the same reasoning as in solving equations.
(Grades
9 -
12)
More Details
Do you agree with this alignment?
International Technology and Engineering Educators Association - Technology
-
Students will develop an understanding of the relationships among technologies and the connections between technology and other fields of study.
(Grades
K -
12)
More Details
Do you agree with this alignment?
-
Students will develop an understanding of the attributes of design.
(Grades
K -
12)
More Details
Do you agree with this alignment?
-
Select resources that involve tradeoffs between competing values, such as availability, cost, desirability, and waste, while solving problems.
(Grades
9 -
12)
More Details
Do you agree with this alignment?
-
Demonstrate the use of conceptual, graphical, virtual, mathematical, and physical modeling to identify conflicting considerations before the entire system is developed and to aid in design decision making.
(Grades
9 -
12)
More Details
Do you agree with this alignment?
-
Optimize a design by addressing desired qualities within criteria and constraints.
(Grades
9 -
12)
More Details
Do you agree with this alignment?
State Standards
Texas - Math
-
solve linear equations in one variable, including those for which the application of the distributive property is necessary and for which variables are included on both sides;
(Grade
9)
More Details
Do you agree with this alignment?
-
create and use representations to organize, record, and communicate mathematical ideas;
(Grade
9)
More Details
Do you agree with this alignment?
Texas - Science
-
Science concepts. The student knows concepts of force and motion evident in everyday life. The student is expected to:
(Grades
9 -
10)
More Details
Do you agree with this alignment?
-
calculate the effect of forces on objects, including the law of inertia, the relationship between force and acceleration, and the nature of force pairs between objects;
(Grades
9 -
12)
More Details
Do you agree with this alignment?
-
develop and interpret free-body force diagrams; and
(Grades
9 -
12)
More Details
Do you agree with this alignment?
Worksheets and Attachments
Visit [www.teachengineering.org/lessons/view/uoh_rocket_lesson01] to print or download.Pre-Req Knowledge
Students should have knowledge and experience with the following concepts: trigonometry, forces, impulse and momentum.
Introduction/Motivation
Hundreds of years ago, the realistic idea of flight did not exist. With the understanding of the basics of flight, inventors determined how to put a human in the air for a few seconds. In the 1960s, President John F. Kennedy proposed that within a decade, we would put a man on the moon; the United States succeeded with the help of a visionary team and aerospace engineers at NASA.
Today, with the Mars rover dominating the news from NASA, have you ever wondered what goes into the planning for such an event? While we might not be able to launch our rocket to Mars, we can explore some of the fundamental concepts that govern such a mission. Essentially, four forces are at play: lift, thrust, gravity and drag. Some forces are more dominate than others, and by understanding what forces are acting on your rocket and the equations governing the forces, you can begin to model the flight of your rocket. Teamwork is essential during this activity, just as it is in professional engineering.
During this lesson, we learn how to perform rocketry calculations. Then, you design your own rocket by choosing an engine and rocket body to launch into the air—as high as the length of a football field!
Lesson Background and Concepts for Teachers
On the first day, students choose rocket parameters and calculate how high their rockets will go after a brief lesson and board activity or pre-assessment of their knowledge. Review the concepts and important parameters in the Rocketry Handout by going through each variable and its unit. Then students may begin completing the Rocket Calculation Worksheet.
On the second class meeting (about one week later), optimize the calculated rocket flight by adjusting the engine size and rocket body, such that results of calculations show that the rockets will fire as close to 100 m as possible (about a football field's length into the air). Student groups submit a Rocky Launch Proposal that includes their rocket and engine selection, calculated height, and mission purpose. Review each proposal before student groups proceed to construction.
During the next class periods, help students address any issues with their calculations. (Note: Allow a full week for completion of the calculations.) Students may have issues with changing the masses into kilograms or changing other units into base SI units. Remind students to make logical calculations. Ask students: can you have a negative or zero height, negative velocity? (No, No, Yes) If you use a smaller rocket and a larger engine, what will happen to the theoretical height? (It will go higher, theoretically.) What effect will a large rocket and a smaller engine have on the theoretical height? (It will go lower, theoretically.)
After initial completion of the calculations, make available a digital spreadsheet to enable students to refine their calculations. (However, inform students that they need not do the same calculations over and over; they should utilize the spreadsheet if they are still having difficulties. (Teacher tip: For ease, post the spreadsheet on Google Drive and share the folder with your students. Alternatively, use Moodle or other shared website. Or, have students access the spreadsheet on a computer in your classroom during class time or after school.)
After completion of the rockets lesson, students begin the associated hands-on activity, Rocket Launch Time: Flying with Style. For the activity finale, student groups launch their rockets. Expect those with the best calculations and care in building their rockets to have the most success. Thus, remind students to be attentive in their calculations, as they impact their rocket performance.
Before the Lesson Worksheet
- As you review the stages of flight, ask students which forces are present and in which direction they are acting. Present to students the Forces and Newton's Laws Presentation, with specific focus on the last three slides, which present free-body diagrams for the different stages a rocket experiences.
- Ask students what parameters they feel are important for design (weight, thrust and cross-sectional area). Weight is important because the rocket will not go as high if it is heavier. Thrust is important because larger engines have larger thrusts causing the rocket to go higher. Cross-sectional area is important because the larger the cross sectional area, the more drag encountered and the less height the rocket attains.
- Discuss which parameters may change with time (mass of propellant, thrust, impulse). The mass of propellant changes with time, as it is burned. The thrust increases as the impulse of the engines provide forward momentum and speed up the rocket during the burn time. Relate this back to the basic design and types of design issues that NASA aerospace engineers must overcome when launching rockets into space, as mentioned in the lesson summary.
- Guide students through the Rocket Calculation Worksheet; be sure to emphasize the use of SI units (kg, N, seconds and meters). Note that the equations are in the order in which they need to be solved. Refer to the Rocket Calculation Worksheet Sample Answer Key as a teacher reference.
- Divide the class into groups of three students each and direct them to get started on their rocket design proposals. (Roles for groups of three are flight director, parts marshal and mission commander. See the Rocketry Handout for more information.)
Teacher Notes
After day one, set a deadline for proposal submission (during week two, or prior to the next rockets lesson). Order rockets based on accurate student calculations (check against class worksheet), build rockets, and set launch date. Allow a month for ordering and delivery of rockets. It is possible for rockets to be built during one class period.
The purpose of posting an Excel spreadsheet online is so students can check their calculations. Encourage them to go through several iterations to try and maximize the height as close to 100 m as possible. If necessary, provide students with additional time during class or before/after school to work on their calculations.
Associated Activities
- Rocket Launch Time: Flying with Style - Student groups construct and launch the rockets that were designed during the lesson. They compare the actual height of the launch to the calculated theoretical height and determine assumptions that lead to differences in the two heights.
Vocabulary/Definitions
action-reaction pairs: Thrust backward of the rocket causes a propulsion forward of the rocket.
applied force : A force that gives the rocket forward acceleration (usually a rocket booster). Thrust is an example.
free-body diagram: A diagram that shows all the forces acting upon an object.
Newton's first law : If the forces are balanced, the body will stay at rest or continue with the same velocity, neither accelerating nor decelerating. Example: A rocket on the launch pad will not move without an outside force.
Newton's second law: If the forces are unbalanced, the body will accelerate in an inverse proportion to the mass and direct proportion to the force. F=ma. Example: A large rocket will require more force or a larger engine for the same acceleration.
Newton's third law: For every action, there is an equal and opposite reaction.
resistive forces : A force that slows the rocket down—usually gravity if on the rise or parachute resistance during free fall. Wind drag is another example.
rocket: A device that has an engine to lift off into the air. The Apollo 11 was the first rocket on the Moon.
Assessment
Pre-Lesson Assessment
Survey/Board Activity – List the vocabulary terms on the classroom board and assess student knowledge about the words and their definitions. Fill in gaps as necessary so that students have enough knowledge to complete the lesson worksheet and select a rocket booster size.
Homework and Lesson Summary Assessment
Rocketry Homework – Have students complete the Rocketry Handout and the Rocket Calculation Worksheet. Use the Rocket Calculation Worksheet Sample Answer Key to assess whether students understand the basic concepts of rocket flight. (Note: It is important that students do not get lost in the equations. Keep them focused on making logical answers so that they can learn to recognize answers that make sense. Reinforce this during the follow-up period when issues are addressed.)
Additional Multimedia Support
Show students photographs or videos of space capsules successfully landing in the ocean, such as this 15-second video: https://www.youtube.com/watch?v=2tyBDB2dFqQ
Subscribe
Get the inside scoop on all things TeachEngineering such as new site features, curriculum updates, video releases, and more by signing up for our newsletter!More Curriculum Like This

The purpose of this lesson is to teach students how a spacecraft gets from the surface of the Earth to Mars. Students first investigate rockets and how they are able to get us into space. Finally, the nature of an orbit is discussed as well as how orbits enable us to get from planet to planet — spec...

Through the continuing storyline of the Rockets unit, this lesson looks more closely at Spaceman Rohan, Spacewoman Tess, their daughter Maya, and their challenges with getting to space, setting up satellites, and exploring uncharted waters via a canoe. Students are introduced to the ideas of thrust,...

Students acquire a basic understanding of the science and engineering of space travel as well as a brief history of space exploration. They learn about the scientists and engineers who made space travel possible and briefly examine some famous space missions.

Students explore motion, rockets and rocket motion while assisting Spacewoman Tess, Spaceman Rohan and Maya in their explorations. First they learn some basic facts about vehicles, rockets and why we use them. Then, they discover that the motion of all objects—including the flight of a rocket and mo...
References
Culp, Randy. http://my.execpc.com/60/B3/culp/. Last updated September 16, 2013. (Used to check the equations that govern the flight of the rocket.)
Estes-Cox Corp, http://www.estesrockets.com/, accessed September 13, 2013. (Source of rocket kits, prices and assembly procedure.)
Other Related Information
Ensure that all of the submitted designs are below the 100-200 meter altitude limit.
Copyright
© 2013 by Regents of the University of Colorado; original © 2012 University of HoustonContributors
Don McGowan; Brian RohdeSupporting Program
National Science Foundation GK-12 and Research Experience for Teachers (RET) Programs, University of HoustonAcknowledgements
This digital library content was developed by the University of Houston's College of Engineering under National Science Foundation GK-12 grant number DGE-0840889. However, these contents do not necessarily represent the policies of the NSF and you should not assume endorsement by the federal government.
Last modified: July 20, 2023
User Comments & Tips