Quick Look
Grade Level: 4 (3-5)
Time Required: 30 minutes
Lesson Dependency: None
Subject Areas: Earth and Space, Physical Science, Science and Technology
NGSS Performance Expectations:
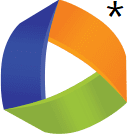
3-PS2-1 |
Summary
One exciting challenge for engineers is the idea of exploration. Through the continuing storyline of the Rockets unit, this lesson looks more closely at Spaceman Rohan, Spacewoman Tess, their daughter Maya, and their challenges with getting to space, setting up satellites, and exploring uncharted waters via a canoe. This lesson reinforces rockets as a vehicle that helps us explore outside the Earth's atmosphere (that is, to move without air) by employing the principles described in Newton's third law of motion. Students are also introduced to the ideas of thrust, control and weight—all principles that engineers deal with when building rockets.Engineering Connection
Designing and building rockets requires many different types of engineers working together to create equipment that works as intended. Engineers must understand Newton's three laws of motion as well as how exhaust behaves in order to calculate the thrust for a rocket to reach its destination. Engineers must also watch the weight of the rocket very carefully because heavier rockets take more energy to get into space. Another important consideration is how to control the rocket. Lastly, understanding how a rocket behaves in the Earth's atmosphere—as well as in space—is critical in rocket design. To enhance stability, engineers include solutions such as fins or small thrusters into their rocket designs.
Learning Objectives
After this lesson, students should be able to:
- Compare and contrast airplanes and rockets and explain why airplanes cannot travel into space.
- Define what thrust is and how rockets use thrust to get into space.
- Explain several considerations engineers have in designing rockets.
- Give an example of an explorer and describe reasons why explorers and exploration teams include engineers.
Educational Standards
Each TeachEngineering lesson or activity is correlated to one or more K-12 science,
technology, engineering or math (STEM) educational standards.
All 100,000+ K-12 STEM standards covered in TeachEngineering are collected, maintained and packaged by the Achievement Standards Network (ASN),
a project of D2L (www.achievementstandards.org).
In the ASN, standards are hierarchically structured: first by source; e.g., by state; within source by type; e.g., science or mathematics;
within type by subtype, then by grade, etc.
Each TeachEngineering lesson or activity is correlated to one or more K-12 science, technology, engineering or math (STEM) educational standards.
All 100,000+ K-12 STEM standards covered in TeachEngineering are collected, maintained and packaged by the Achievement Standards Network (ASN), a project of D2L (www.achievementstandards.org).
In the ASN, standards are hierarchically structured: first by source; e.g., by state; within source by type; e.g., science or mathematics; within type by subtype, then by grade, etc.
NGSS: Next Generation Science Standards - Science
NGSS Performance Expectation | ||
---|---|---|
3-PS2-1. Plan and conduct an investigation to provide evidence of the effects of balanced and unbalanced forces on the motion of an object. (Grade 3) Do you agree with this alignment? |
||
Click to view other curriculum aligned to this Performance Expectation | ||
This lesson focuses on the following Three Dimensional Learning aspects of NGSS: | ||
Science & Engineering Practices | Disciplinary Core Ideas | Crosscutting Concepts |
Science explanations describe the mechanisms for natural events. Alignment agreement: | Each force acts on one particular object and has both strength and a direction. An object at rest typically has multiple forces acting on it, but they add to give zero net force on the object. Forces that do not sum to zero can cause changes in the object's speed or direction of motion. (Boundary: Qualitative and conceptual, but not quantitative addition of forces are used at this level.) Alignment agreement: Objects in contact exert forces on each other.Alignment agreement: | Cause and effect relationships are routinely identified. Alignment agreement: |
International Technology and Engineering Educators Association - Technology
-
Students will develop an understanding of the relationships among technologies and the connections between technology and other fields of study.
(Grades
K -
12)
More Details
Do you agree with this alignment?
-
The use of transportation allows people and goods to be moved from place to place.
(Grades
3 -
5)
More Details
Do you agree with this alignment?
Introduction/Motivation
Tess and Maya are joining a long line of explorers who have explored many parts of the world. Can you name any explorers and/or the parts of the world that they have explored? What sort of engineering teams do you think they needed to be successful? Listed below are some explorers who were engineers or needed engineering-designed equipment to achieve their exploration goals:
Sylvia Earl: In 1970, Sylvia Earl and four other women dove 50 feet below the ocean's surface and lived in a small structure for two weeks. In 1979, she walked untethered on the sea floor at a lower depth than any living human being before or since. She wore a "Jim" suit—a pressurized one-atmosphere garment, and was carried by a submersible down to a depth of 1,250 feet below the ocean's surface (Academy of Achievement, "Ambassador for the World's Oceans: Sylvia Earl, Underwater Explorer," http://www.achievement.org/autodoc/page/ear0bio-1). (What type of engineering did her underwater feat involve? Possible answers: Design of the "Jim" suit, the underwater structure, the submersible, etc.)
Junko Tabei, Ming Kipa, Temba Tshiri: On May 16, 1975, Junko Tabei of Japan became the first woman to reach the summit of Mount Everest (Steponline: Everyone Has an Everest, "Junko Tabei: The first woman to summit Everest"). To date, the youngest person to summit Everest is female climber Ming Kipa who summited Everest in 2003 at age 15. The earlier record was held by Temba Tshiri, a Nepalese Sherpa, who at the age of 16 years and 17 days became the youngest person to ever scale Everest on May 23, 2001 (http://www.adventurestats.com/tables/everestage.htm). (What type of engineering did these explorers involve? Possible answers: Design of climbing equipment, eye protection, tent and warm clothing)
Amelia Earhart: In 1932, this "First Lady of the Air" became the first woman to fly solo across the Atlantic Ocean. Fifteen hours and 19 minutes after she left Harbour Grace, Newfoundland, she landed her flame-shooting plane in a farmer's field in Londonderry, Ireland (http://kids.niehs.nih.gov/science/quizzes/american_women_quiz.htm). (What engineering did Earhart utilize? Possible answers: Design of the plane and its controls.)
Sacagewea: This Native-American explorer whose name means "Bird Woman" served as a guide to Lewis and Clark on their expedition to the western U.S. in 1804. She knew how to survive living off the land, enabling the expedition's success in reaching the Pacific coast (http://kids.niehs.nih.gov/science/quizzes/american_women_quiz.htm). (What type of engineering? Possible answer: Sacagewea would have been an engineer herself, navigating the land in a way to help her survive. Early navigators helped influence the technical navigation equipment used today.)
Sally Ride: She was the first American woman to watch 16 sunrises and 16 sunsets every 24 hours. In June 1983, she became the youngest flyer and the first American woman to rocket into space. For more than six days, she served as the flight engineer for the space shuttle Challenger, launching and retrieving satellites (http://kids.niehs.nih.gov/science/quizzes/american_women_quiz.htm). (What type of engineering did Ride's exploration involve? Possible answers: She was the flight engineer for the mission, engineers designed the space shuttle and satellites.)
Valentina Vladimirovna Tereshkova: This colonel-engineer and Soviet cosmonaut became the first woman in space. She was on the Vostok 5 mission which launched on June 16, 1963, and orbited the Earth 48 times. The flight lasted 2.95 days (70.8 hours). During her space mission, Tereshkova's radio call name was "Chaika," which means "seagull" in Russian (http://www.allaboutspace.com/explorers/page/t/tereshkova.shtml). (What type of engineering did this space flight involve? Possible answers: Valentina was an engineer on this mission, and engineers designed the rocket.)
You all, as members of Spaceman Rohan's, Spacewoman Tess', and Maya's engineering team, are joining a lengthy and accomplished list of engineers who have helped design and build exploration vehicles. For Tess to get herself and her satellites into space, she needs you as her engineering team to figure out how exactly to send equipment (and Tess!) into space successfully.
Can an airplane just fly into space? No it cannot! Most people know that airplanes cannot fly in space, but few really know why. The answer: airplanes need air to fly, and there is no air in space. Airplanes have certain parts that help them fly through the air. What does a propeller do and are they ever used on a rocket? (Answer: Hopefully they are never used on a rocket.) Propellers have angled blades that push air—like a fan does. Airplanes also have wings that use air to help them stay in flight. Rockets are different from airplanes because rockets fly into space. With no air in space, rockets cannot make use of propellers or wings.
How do engineers get rockets into space? Remember Newton's third law of motion about equal and opposite forces? We know that objects can be moved in one direction by ejecting mass in the opposite direction. Is getting a rocket into space just as simple as the third law sounds? No! Rockets are big and heavy! (See Figure 1.) It takes a huge force to launch rockets into space. To make things even more difficult, rockets do not just fly straight on their own.
Engineers must consider three main things when designing rockets that can be launched into space include: thrust, weight and control. Thrust directly relates to Newton's third law, which tells us how rockets move—by pushing out mass in one direction to move in the opposite direction (Refer to the associated activity Strawkets and Thrust for students to observe the significance of this variable in rocket design). In some rockets, the thrust comes from pushing out hot gases that come from burning fuel. These rockets move as far up as the force of the gas fuel pushes down. How do you think weight affects a rocket? Sudents can further investigate the relationship with the associated activity Strawkets and Weight. Well, the heavier the rocket weighs, the more thrust it needs to take off. Weight is very important to engineers trying to determine how to get a rocket into space. Lastly, engineers have to work on rocket control to get a rocket to move in the right direction. Control is important for getting the rocket past the Earth's atmosphere safely. Engineers must specifically design rockets to be balanced and stable while flying. Imagine you are riding in a rocket to outer space. (Refer to the associated activity Strawkets and Control to investigate the importance of stability in rocket design) How would you feel if your rocket started shaking and moving uncontrollably? Today we will learn some basic concepts of rocket engineering so that this scary situation does not occur.
Lesson Background and Concepts for Teachers
Thrust
Rockets move by expelling mass (action) in one direction to move the rocket in the opposite direction (reaction). This is called a thrust force and is described by Newton's third law of motion.
With chemical rockets such as the ones seen on the Space Shuttle, the action is the expelling of hot gas propellant out of the engine. This causes movement of the rocket, a reaction, as the rocket moves in the opposite direction. To enable a rocket to lift off from the launch pad, the action—or thrust—from the engine must be greater than the force from the downward acceleration of gravity on the mass of the rocket.
Rockets work better in space than they do in air! While in the Earth's atmosphere, a rocket moves slower because of the surrounding air. This phenomenon, called drag, is due to air resistance. Air resistance can be demonstrated using a piece of paper. If you hold a piece of paper flat above the floor and drop it, the paper slowly floats down to the floor. However, if you crumple up the same piece of paper and drop it, it falls to the floor much more quickly. The crumpled paper experiences less drag because it has a smaller surface area. In other words, the air does not have as large of an area to resist the motion of the falling paper. At the point when rockets get to space, they no longer encounter any any air resistance to slow them down.
To get more thrust out of slow-moving rockets (such as paper rockets), the exit "nozzle" must be as small as possible to make the air accelerate faster (see Figure 2).
Because it is a gas, air can be compressed. Devices called air compressors use a pump to push air into a rigid container, storing a tremendous amount of air in a small space at very high pressure. The pump uses a large force to compress the air. When the air is released from the container, it generally blows out very hard and very fast (such as when you user a compressor to fill bicycle or car tires). When air moves relatively slowly (~200 mph or less) through a tube that is decreasing in diameter, it does not compress. Under these conditions, not enough force is exerted on the air to make it squeeze together. Instead, the air speeds up. This is what happens when you open a door to your home or a building. If you are standing next to the door inside the house/building, you feel a breeze since the air speeds up as it moves through the doorway. Another example is water flowing through a garden hose. If you place your thumb over the end of the hose to partially block the water from exiting, the water sprays out much faster than if you let it flow without blockage. When the water has nowhere to go, its only option is to speed up.
But why then do pictures of rockets usually show bell-shaped nozzles (see Figure 3)? Why would engineers make the nozzle area larger? Based on what we have learned, wouldn't that mean that the hot gases coming out would move slower? No, rockets usually travel faster than the speed of sound and that changes how air behaves in a nozzle. Once air is moving faster than the speed of sound, it behaves differently than on Earth! When air moves this fast, it becomes compressible. It will slow down if the nozzle diameter gets smaller (see Figure 4). This explains the bell shape for rocket nozzles. In order for the hot gases to then speed up, the shape of the nozzle must expand (see Figure 5).
Weight
The weight of a rocket is the force that opposes motion and thrust. A heavier rocket requires more thrust to reach space, which ultimately increases the overall cost of the rocket. Engineers try to find ways to reduce the weight of a rocket by using lightweight materials. However, lighter is not always better. For example, a rocket made of paper would not survive a launch into space since it lacks the necessary structural integrity to endure the harsh elements of space and the forces encountered in space flight. Furthermore, strong, yet lightweight materials are often expensive to design and fabricate. A good engineer must come up with a solution that balances each of these competing needs.
Fun Fact: The weight of one solid rocket booster of the space shuttle (it has two total) is 1,252,000 pounds, including the propellant! (source: http://www.atk.com/rocketmotors/rocketmotors_rsrm.asp).
The weight distribution of a rocket is also important. The point about which a rocket's weight is equally balanced is called its center of gravity (CG). The concept of center of gravity can be demonstrated by balancing a meter stick horizontally on your finger placed at the 50 cm mark. The meter stick's CG is located at the 50 cm mark because all of the weight on one side of the mark is equal to the weight on the other side of the mark.
Control
The motion of any naturally unstable object is almost impossible to predict. For example, if you pick up a football and throw it without the proper grip and technique, it wobbles through the air and is difficult for someone on the receiving end to catch. A quarterback spirals a football is so that it completes a controlled trajectory, making it easy for a receiver to decide where to place his hands. Why does an untied balloon fly all over the place when let go? It has no control. The opening from which the air escapes is flexible and the round shape of the balloon means it can rotate in any direction as it pushes through the air.
It is necessary to control a rocket in the atmosphere before it reaches space. We know that airplanes have wings, but they usually also have smaller fins on the tail as well. Why do you think that similar small fins are often seen on rockets?
Wings only provide lift perpendicular to the motion of an object, and they create drag—which is okay for airplanes flying horizontally (so lift is generated vertically). Rockets, however, are trying to get to space, and the most efficient way to do that is by heading straight up with as little drag as possible. Rockets do not need lift from wings. Instead they get all of their lift from engine thrust. The smaller fins help provide the necessary control a rocket needs immediately after lift off (or launch).
The trade-off between wing lift and drag is shown in Figure 6. If an object with wings has a constant thrust (like an airplane), it can stay aloft longer. The extra thrust counteracts the drag and keeps the wings generating lift. Now, look at the wing with no extra thrust. At first it seems better than a rocket with fins, but the wing creates a lot of drag; however, without the extra thrust to compensate, all the forward motion is lost, which decreases the lift and gravity quickly pulls it down! Small fins do not generate lift, but they more than make up for it by not adding much weight or drag.
Since rockets fly through the air, another important point must be considered in addition to the rocket's center of gravity (CG): the center of pressure (CP). The CP is different from the CG in that it is the point about which the surface area of the rocket is equally balanced on both sides, not the weight. For a stable rocket, the CP should be behind the CG—meaning if the rocket is pointed upward, the CP should be below the CG. This can be accomplished by either adding fins near the back of the rocket (which increases surface area in the back and thus moving the CP back) or adding mass near the front of the rocket (which moves the CG up further towards the top). However, if the center of gravity and the center of pressure are at the exact same spot, the object rotates randomly in either direction at any time. Engineers must always decide where to locate these two points on a rocket, and in relation to each other, in order to design the best rocket possible.
Associated Activities
- Strawkets and Thrust - Students observe how to increase thrust by making their own paper rockets.
- Strawkets and Weight - Students test paper rockets of varying weights.
- Strawkets and Control - Students investigate how to increase rocket stability.
Lesson Closure
Today, we learned more about rockets and how they are different from airplanes. Who can remember why rockets are different than airplanes? (Answer: Airplanes need air to fly; in space, rockets must fly without air.) How do engineers get rockets into space? Which law was that? (Answer: Newton's third law of motion.) From Newton's third law, we know that objects can be moved in one direction by ejecting mass in the opposite direction. Who remembers three things that engineers must consider when designing rockets that will be launched into space? (Answer: Thrust, weight and control.) What is thrust? (Answer: Thrust is the force on a rocket that moves it in a forward direction. In some rockets, the thrust comes from pushing out hot gases from burning fuel.) We learned the importance of rocket thrust, weight and control. Now we can use what we have learned to understand how engineers design real rockets!
As Spaceman Rohan and Spacewoman Tess' engineering team, you now better understand how to build Tess' rocket. You are also following in the footsteps of many engineering teams who have helped (and have been) explorers that continue to explore our world and beyond.
Vocabulary/Definitions
center of gravity (CG): The average location of the weight of an object (that is, the balance point).
center of pressure (CP): The average location of the pressure variation over an object. (For a sailboat, this would be near the center of the sail.)
drag: Friction force opposing an object's motion (in this lesson, air causes the friction). It is generated by the interaction and contact of a solid body with a fluid (liquid or gas).
fin: A small, flat surface that uses airflow to stabilize a rocket. Fins offer no lift and have very little drag.
perpendicular: Something that lies at a 90° angle.
rocket: A vehicle that moves by ejecting mass.
trajectory: The path an object travels through space.
wing: A flat or curved surface that creates a pressure difference producing lift and drag.
Assessment
Pre-Lesson Assessment
Cooperative Group Brainstorming: Organize students in groups of four. Ask them to write down everything they know about rockets and report to the class how they think rockets actually get into space and if they are heavy or light. Have them draw a sketch of a rocket (any one that comes to mind) and have them explain how the different parts of the rocket might be used.
Venn Diagram: Have students create a class venn diagram or T-chart to compare what they know about airplanes and rockets.
Post-Introduction Assessment
Question/Answer: Ask the students and discuss as a class:
- Why do explorers and exploration teams utilize engineers? (Answer: Engineers design the equipment that helps explorers and teams conduct successful missions. Engineers have helped, designed, built and used most exploration vehicles.)
- How are airplanes different than rockets? (Answer: Airplanes have wings and sometimes propellers to help them fly through the air. There is no air in space, so rockets cannot use the same parts as airplanes.)
- What are three things that engineers need to consider when designing a rocket that can get into space? (Answer: weight, thrust and control)
- How do rockets use thrust to get into space? (Answer: Thrust directly relates to Newton's third law of motion. It tells us how rockets move into space by pushing out fuel in one direction to move in the opposite direction.)
- Can you change the weight of a rocket without changing the thrust and control? (Answer: No, all three factors are interrelated.)
- On an actual rocket, if you add propellant (fuel) to get more thrust, what other key factor have you affected? (Answer: The weight; fuel is heavy.)
Lesson Summary Assessment
Cooperative Group Brainstorming Revisited: Pass back the papers that were used in the Pre-Lesson Assessment. In the same groups, have the students read over their answers. Ask them to update their answers, and if they want to, sketch another rocket on a new sheet of paper. Then, call on volunteers to make a brief presentation to the class about what they thought before the lesson and what they have learned now.
Interesting Facts/Discussion Questions: Solicit, integrate and summarize student responses.
- Do any human-made vehicles fly through both air and space? (Answer: Yes, the space shuttle does both, but it really only glides in the atmosphere. Right now, no vehicle exists that takes off like an airplane and can make it to space, but engineers are currently working on this.)
- Spacecraft can escape the Earth's gravity and travel to other planets. Can a spacecraft escape the sun's gravity and leave the solar system? (Answer: Yes, the Voyager spacecraft has already done that.)
Lesson Extension Activities
Have students create T-charts to compare model rockets to real rockets. Use this following website as a discussion guide: http://exploration.grc.nasa.gov/education/rocket/rktcompare.html
Have students investigate additional ways that engineers control rockets besides the use of fins. A good place to start is http://exploration.grc.nasa.gov/education/rocket/rktcontrl.html
Have students research the weight and required thrust of some of NASA's rockets.
Subscribe
Get the inside scoop on all things TeachEngineering such as new site features, curriculum updates, video releases, and more by signing up for our newsletter!More Curriculum Like This

Students study how propellers and jet turbines generate thrust. This lesson focuses on Isaac Newton's third law of motion for every action there is an equal and opposite reaction.

The purpose of this lesson is to teach students how a spacecraft gets from the surface of the Earth to Mars. Students first investigate rockets and how they are able to get us into space. Finally, the nature of an orbit is discussed as well as how orbits enable us to get from planet to planet — spec...

Students explore motion, rockets and rocket motion while assisting Spacewoman Tess, Spaceman Rohan and Maya in their explorations. First they learn some basic facts about vehicles, rockets and why we use them. Then, they discover that the motion of all objects—including the flight of a rocket and mo...

Students acquire a basic understanding of the science and engineering of space travel as well as a brief history of space exploration. They learn about the scientists and engineers who made space travel possible and briefly examine some famous space missions.
References
Academy of Achievement, "Ambassador for the World's Oceans: Sylvia Earl, Underwater Explorer." Accessed January 2006. http://www.achievement.org/autodoc/page/ear0bio-1
Alliant Techsystems Inc. "RSRMs — Reusable Solid Rocket Motors." Accessed March 1, 2012. www.atk.com
Col, Jeananda. Enchanted Learning, "Valentina Vladimirovna Tereshkova: The First Woman in Space." Accessed March 1, 2012. www.allaboutspace.com/explorers/page/t/teresahkova.shtml
MountEverest.net: by climbers. Accessed March 2, 2012. www.explorersweb.com/everest_k2/
NIEHS Office of Management, The NIEHS Kids' Pages, "American Women: From Adventure to Invention," National Institute of Environmental Health Sciences. Accessed January 2006. http://www.niehs.nih.gov/
Steponline: Everyone Has an Everest, "Junko Tabei: The first woman to summit Everest." Accessed January 18, 2006. http://www.steponline.com/everest.junko_tabei.asp
Copyright
© 2006 by Regents of the University of ColoradoContributors
Jeff White; Brian Argrow; Luke Simmons; Jay Shah; Malinda Schaefer Zarske; Janet YowellSupporting Program
Integrated Teaching and Learning Program, College of Engineering, University of Colorado BoulderAcknowledgements
The contents of this digital library curriculum were developed under grants from the Fund for the Improvement of Postsecondary Education (FIPSE), U.S. Department of Education and National Science Foundation (GK-12 grant no. 0338326). However, these contents do not necessarily represent the policies of the DOE or NSF, and you should not assume endorsement by the federal government.
Last modified: January 28, 2021
User Comments & Tips