Quick Look
Grade Level: 10 (9-12)
Time Required: 45 minutes
Expendable Cost/Group: US $0.00 This activity requires some non-expendable (and reusable) items for each group. See Materials List for details.
Group Size: 3
Activity Dependency:
Subject Areas: Measurement, Physical Science, Science and Technology
NGSS Performance Expectations:
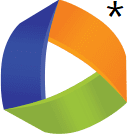
HS-ESS3-4 |
Summary
Student teams measure voltage and current in order to determine the power output of a photovoltaic (PV) panel. They vary the resistance in a simple circuit connected to the panel to demonstrate the effects on voltage, current, and power output. After collecting data, they calculate power for each resistance setting, creating a graph of current vs. voltage, and identifying the maximum power point.Engineering Connection
Photovoltaic (PV) panels utilize a scientific technology that creates power from solar radiation. Because PV panels are expensive and their power production is limited by the amount of sunlight available, it is important for them to run as efficiently as possible. One way to improve PV panel efficiency is to adjust the resistance in the design of the electrical circuit to create a combination of voltage and current that results in the greatest power output. Engineers must understand how to control a basic circuit in order to design PV arrays that operate as efficiently as possible.
Learning Objectives
After this activity, students should be able to:
- Measure the voltage and current of a photovoltaic (PV) electrical circuit.
- Explain how to calculate and maximize the DC power output of a PV system.
Educational Standards
Each TeachEngineering lesson or activity is correlated to one or more K-12 science,
technology, engineering or math (STEM) educational standards.
All 100,000+ K-12 STEM standards covered in TeachEngineering are collected, maintained and packaged by the Achievement Standards Network (ASN),
a project of D2L (www.achievementstandards.org).
In the ASN, standards are hierarchically structured: first by source; e.g., by state; within source by type; e.g., science or mathematics;
within type by subtype, then by grade, etc.
Each TeachEngineering lesson or activity is correlated to one or more K-12 science, technology, engineering or math (STEM) educational standards.
All 100,000+ K-12 STEM standards covered in TeachEngineering are collected, maintained and packaged by the Achievement Standards Network (ASN), a project of D2L (www.achievementstandards.org).
In the ASN, standards are hierarchically structured: first by source; e.g., by state; within source by type; e.g., science or mathematics; within type by subtype, then by grade, etc.
NGSS: Next Generation Science Standards - Science
NGSS Performance Expectation | ||
---|---|---|
HS-ESS3-4. Evaluate or refine a technological solution that reduces impacts of human activities on natural systems. (Grades 9 - 12) Do you agree with this alignment? |
||
Click to view other curriculum aligned to this Performance Expectation | ||
This activity focuses on the following Three Dimensional Learning aspects of NGSS: | ||
Science & Engineering Practices | Disciplinary Core Ideas | Crosscutting Concepts |
Design or refine a solution to a complex real-world problem, based on scientific knowledge, student-generated sources of evidence, prioritized criteria, and tradeoff considerations. Alignment agreement: | Scientists and engineers can make major contributions by developing technologies that produce less pollution and waste and that preclude ecosystem degradation. Alignment agreement: When evaluating solutions it is important to take into account a range of constraints including cost, safety, reliability and aesthetics and to consider social, cultural and environmental impacts.Alignment agreement: | Feedback (negative or positive) can stabilize or destabilize a system. Alignment agreement: Engineers continuously modify these technological systems by applying scientific knowledge and engineering design practices to increase benefits while decreasing costs and risks.Alignment agreement: |
Common Core State Standards - Math
-
Represent data on two quantitative variables on a scatter plot, and describe how the variables are related.
(Grades
9 -
12)
More Details
Do you agree with this alignment?
-
For a function that models a relationship between two quantities, interpret key features of graphs and tables in terms of the quantities, and sketch graphs showing key features given a verbal description of the relationship.
(Grades
9 -
12)
More Details
Do you agree with this alignment?
International Technology and Engineering Educators Association - Technology
-
Energy resources can be renewable or nonrenewable.
(Grades
9 -
12)
More Details
Do you agree with this alignment?
-
Power systems must have a source of energy, a process, and loads.
(Grades
9 -
12)
More Details
Do you agree with this alignment?
State Standards
Colorado - Math
-
Graph linear and quadratic functions and show intercepts, maxima, and minima.
(Grades
9 -
12)
More Details
Do you agree with this alignment?
-
Solve linear equations and inequalities in one variable, including equations with coefficients represented by letters.
(Grades
9 -
12)
More Details
Do you agree with this alignment?
Colorado - Science
-
Use appropriate measurements, equations and graphs to gather, analyze, and interpret data on the quantity of energy in a system or an object
(Grades
9 -
12)
More Details
Do you agree with this alignment?
-
Use direct and indirect evidence to develop and support claims about the conservation of energy in a variety of systems, including transformations to heat
(Grades
9 -
12)
More Details
Do you agree with this alignment?
Materials List
Each group needs:
- mini PV panel ($10-30; available online; do a product search for "small solar panel" or see the Solar Panel Source Information attachment in the Photovoltaic Efficiency unit)
- 4 to 5 wires with alligator clips
- 1 or 2 multimeters ($10; available online; see the Solar Multimeter Source Information attachment in the Photovoltaic Efficiency unit) (experiment can be run with either one or two multimeters per group, see Procedure section for details)
- 10K Ohm potentiometer ($2; available online at amazon.com, for example at https://www.amazon.com/Parts-Express-10K-Ohm-Potentiometer/dp/B0002KRE20) Note: the resistance needed depends on the panel used and the experimental set-up conditions. A 5K Ohm potentiometer was used in the example in the associated lesson. A 10K Ohm potentiometer provides more flexibility, but gives less range of motion until the open circuit voltage is reached.
- sunlight, or a clamp or desk lamp with 100W incandescent bulb (~$8 available at hardware stores; best for each group to have their own, but teams can share if necessary)
- Investigation Worksheet, one per person
- Student Investigation Guide, if using one multimeter per group, OR Student Investigation Guide – Two Multimeters, if using two multimeters per group
Note: The non-expendable items (mini PV panels, multimeters, potentiometer, wires with alligator clamps, lamp and light bulb) are reusable for the entire four-lesson unit, as well as other projects.
Worksheets and Attachments
Visit [www.teachengineering.org/activities/view/cub_pveff_lesson03_activity1] to print or download.Pre-Req Knowledge
A basic understanding of an electrical circuit, including voltage, current, power and resistance.
Introduction/Motivation
(Write the following equations on the board or post somewhere in the classroom.)
Ohm's law: V=I*R
Electrical power equation: P=V*I
where
V = potential difference (in volts or V)
I = current (in amperes or A)
R = resistance (in Ohms or Ω)
P = power (in watts or W)
Photovoltaic (PV) power is a clean and renewable energy source that is gaining popularity and is predicted to become a cost-effective source of electricity. To maximize the offset of greenhouse gases and minimize the long-term cost (maximize the return on investment) for PV panels, engineers must be sure that they can design solar systems that generate the maximum amount of power in all conditions.
Did you know that PV panels do not always produce the same amount of power? For example, the current produced by a PV panel varies depending on the angle between the PV panel and the sun (as demonstrated in A New Angle on PV Efficiency activity). A PV panel that faces the sun receives more direct solar radiation than one that does not, and this helps to maximize the current flowing through the panel circuit. Although positioning has a great impact on the power output of PV panels, it is not the only factor that determines the amount of power generated. Can you think of another factor in our circuit that might affect power? (Possible answers: Voltage, resistance.) The electrical equations (point to the board or posted location in the classroom) show us that power (P) is equal to voltage (V) multiplied by current (I), and also that voltage, current, and resistance are all related by Ohm's law. Engineers use these fundamental electrical relationships to be sure that the circuit bringing power from the panel is designed to maximize the power output of the panel at all times.
Today, we'll be working with our PV panels again. We will record the current and voltage of our PV circuit using a potentiometer that varies the resistance in the circuit, and calculate the resulting power output. If we can find the point with the highest power output, then we have found the maximum power point and we know our panel is running as efficiently as possible for the existing conditions!
Procedure
Background
This procedure describes two ways to conduct this experimental activity: Use the first set-up if you have one multimeter per group; use the second if you have two multimeters per group. The two-multimeter procedure enables faster data collection and more accurate results by eliminating the errors caused by changes in available light and the undesired movement of the materials. But, either experimental set-up works just fine.
The experiment can be conducted either outdoors in sunlight, or indoors using a 100-watt incandescent lamp. Simply place the PV panel under the lamp. This lamp can become extremely hot! Be careful! While conducting the experiment, make sure nothing blocks the light from reaching the panel. Try not to modify the position or any other variables that might disrupt the results.
Before the Activity
- Gather materials and make copies of the Investigation Worksheet.
- If using one multimeter per group set-up, make copies of the Student Investigation Guide. If two multimeters are available per group, make copies of the Student Investigation Guide – Two Multimeters.
- Practice setting up the experiment before running the activity with the students.
- Write the associated equations (Ohm's law and electrical power equation) on the board.
- Also write on the board the symbols for AC (~) and DC ( -- ) and indicate that the DC setting should always be used for this experiment. (As needed, explain the difference between alternating and direct current. This information can be found either in the multimeter manual or online.)
- Show an example set-up of the activity. Use the diagrams in Figures 1-3, as needed.
- Divide the class into groups of three students each.
With the Students: Experiment Procedure (One-Multimeter Set-Up)
- Hand out to each student a worksheet to fill out during and after the activity.
- Hand out to each group a Student Investigation Guide to follow along with the procedure.
- Have each group bring to its work area the necessary experiment supplies (as listed in the Materials List in the guide, including one multimeter). Then have teams conduct steps 4-9.
- Turn the potentiometer dial clockwise until it stops.
Figure 1. The electrical circuit configuration to measure voltage. - Assemble the circuit, as shown in Figure 1 (and in the guide).
- Turn the multimeter to volts DC (DCV) and measure the voltage. Record this value in the worksheet table. (Note: If a negative value displays, then switch the leads.)
- Reconfigure the circuit so that it looks like the diagram provided in Figure 2 (and in the guide).
- Change the multimeter to read amps DC (DCA) and measure the current. Record this value in the worksheet table.
Figure 2. The electrical circuit configuration to measure current. - Turn the potentiometer counterclockwise, slowly, in small increments, until the current in the circuit is approximately 0. Note this position; it will be your furthest turning point. The goal is to get 20 evenly-spaced readings, so ideally you will attempt to turn the potentiometer 1/20th of its turning potential for each reading. (Note: If the potentiometer reaches the turning limit before 20 voltage and current readings have been taken in the experiment, use evenly-spaced clockwise turns to take the final readings.)
- Repeat steps 4-9 and record the data for each turn of the potentiometer.
- After all the data has been collected, return the equipment and clean-up your work area.
With the Students: Experiment Procedure (Two-Multimeter Set-Up)
- Hand out to each student a worksheet to fill out during and after the activity.
- Hand out to each group a Student Investigation Guide – Two Multimeters to follow along with the procedure.
- Have each group bring to its work area the necessary experiment supplies (as listed in the Materials List in the guide, including two multimeters).
- Turn the potentiometer dial clockwise until it stops.
Figure 3. The electrical circuit configuration to measure voltage and current (using two multimeters). - Assemble the circuit, as shown in Figure 3 (and in the guide). Note: Figure 4 provides a photograph of the equipment connected into the experimental set-up with a circuit board; the circuit board you see is not needed if wires with alligator clips are used to connect the equipment.)
Figure 4. Example experimental set-up with circuit board and two multimeters. - Turn the multimeter that measures voltage to volts DC.
- Turn the multimeter that measures current to amps DC
- Measure the voltage and record this value on the worksheet.
- Measure the current and record this value on the worksheet.
- Turn the potentiometer counterclockwise, slowly, in small increments, until the current in the circuit is approximately 0. Note this position; it will be your furthest turning point. The goal is to get 20 evenly-spaced readings, so ideally you will attempt to turn the potentiometer 1/20th of its turning potential for each reading. (Note: If the potentiometer reaches the turning limit before 20 voltage and current readings have been taken in the experiment, use evenly-spaced clockwise turns to take the final readings.)
- Repeat steps 8-10, recording the data for each turn of the potentiometer.
- After all the data has been collected, return the equipment and clean-up your work area.
After Data Collection
- Give students time to complete the post-experiment assignment described on the worksheet. (If time does not allow for in-class completion, make this a homework assignment.) Students are asked to calculate the power of each trial and record it in the table. Then they graph current and power vs. voltage, and identify the maximum power point on their graphs.
- Conclude with a class discussion to review worksheet results. Include post-activity discussion questions provided in the Assessment section.
Vocabulary/Definitions
efficiency: The ratio of the useful energy delivered by a dynamic system to the energy supplied to it.
maximum power point (MPP): The point on a power (I-V) curve that has the highest value of the product of its corresponding voltage and current, or the highest power output.
photovoltaic cell: A cell of silicone that produces a current when exposed to light.
potentiometer: A device that allows the user to vary the electrical resistances in a circuit.
Assessment
Pre-Activity Assessment
Discussion Questions: Ask the students and discuss as a class:
- Do you think a PV panel produces the same amount of power in different weather conditions? Why or Why not?
- What other conditions might change the power output of a PV panel?
- Why is it important to maximize the power output of PV panels?
Activity Embedded Assessment
Activity Worksheet: Have students record measurements and follow along with the activity using the Investigation Worksheet, which includes a chart for recording measured data and power calculations. If time does not allow for in-class completion of the post-experiment power calculations and graphing, make finishing up the worksheet a homework assignment. Review their answers to gauge their mastery of the subject.
Post-Activity Assessment
Discussion Questions: Ask the students the following questions and discuss as a class. See the answers provided in the Investigation Worksheet Answers.
- Would it be more efficient for a large field of PV panels (such as the one shown in Figure 5 and on page 1 of the guide) to have one MPP tracker for the entire field, or to use many MPPTs for smaller areas of the field? Why or Why not?
- What was the maximum power produced by your panel?
- What is the short circuit current (Isc, or current when V=0), and open circuit voltage (Voc, or voltage when I=0) of your PV circuit?
Figure 5. This field of PV panels in Alamosa, CO, is one of the largest PV power plants in the US.
Designing for the Weather: Have students think about the following scenario and write a paragraph about how they would change their design based on the new weather conditions. (This question is also on the worksheet.)
- If a cloud covered your panel and lowered the current in the circuit, what would happen to the maximum power point? Would it be necessary to adjust the resistance to find a new MPP? (Example answer: If a cloud covered a panel, the current would drop immediately and drastically, creating a new shape for the I-V curve. The MPP would drop to a lower value and it would be necessary to adjust the resistance to find the new voltage value, which results in the MPP for the new circuit conditions.)
Safety Issues
- 100-watt incandescent lamps can become extremely hot! Use caution when handling them.
Troubleshooting Tips
The wire connections are very important. Double check to make sure you have tight connections throughout the experimental set-up.
Be sure that the wire ends are not touching any other conductive materials such as metal tables.
The panels do not work well under fluorescent lights due to the reduced light spectrum of those bulbs.
If the multimeter displays negative numbers, switch the leads.
To achieve the best results, do not move equipment, so conditions are kept constant throughout the experiment.
Activity Extensions
Field of PV Panels: Have each group join with another to combine their panels in series and repeat the procedure. Then, have them combine the panels in parallel and repeat the procedure. What are the differences in the current and voltage? (Answers: Adding the panels in series creates a higher voltage. Adding the panels in parallel creates a higher current. Each configuration is useful for specific situations. A disadvantage of series connections is that if one panel is shaded, its lower current output will lower the power output of all panels. This happens often in neighborhoods where a tree is near a solar array. One solution is to use an inverter for each panel, but this would be very expensive. Another solution is to wire each panel to the main inverter separately, which takes more labor. Usually, for large arrays, both series and parallel connections are used to create the necessary voltage, or to isolate inefficiencies caused by shading or problems with single panels.)
Activity Scaling
- For lower grades, conduct the experiment as a class demonstration using a small buzzer. Put the panel in the sun or under the 100-watt lamp, connect the buzzer, and listen as the volume changes when the resistance in the circuit is changed. It may not be exactly clear from the buzzer volume when the panel is at the maximum power point, but explain how the potentiometer changes the resistance in the circuit and this affects the voltage and current, which determines the power output. Buzzers are inexpensive ($4) and can be found at electronics and hardware stores such as RadioShack or online at http://scientificsonline.com/ .
- For upper grades, instead of by hand, have students use a computer and Microsoft Excel software to graph the current and power vs. voltage using their measured data and power calculations (see the post-experiment assignment on the worksheet).
Subscribe
Get the inside scoop on all things TeachEngineering such as new site features, curriculum updates, video releases, and more by signing up for our newsletter!More Curriculum Like This

Students learn how to find the maximum power point (MPP) of a photovoltaic (PV) panel in order to optimize its efficiency at creating solar power. They also learn about real-world applications and technologies that use this technique, as well as Ohm's law and the power equation, which govern a PV pa...

Students learn about current electricity and necessary conditions for the existence of an electric current. Students construct a simple electric circuit and a galvanic cell to help them understand voltage, current and resistance.

Students explore how the efficiency of a solar photovoltaic (PV) panel is affected by the ambient temperature. They learn how engineers predict the power output of a PV panel at different temperatures and examine some real-world engineering applications used to control the temperature of PV panels.

Students learn about the daily and annual cycles of solar angles used in power calculations to maximize photovoltaic power generation. They gain an overview of solar tracking systems that improve PV panel efficiency by following the sun through the sky.
Copyright
© 2009 by Regents of the University of Colorado.Contributors
Stephen Johnson, William Surles, Jack Baum, Abby Watrous, Eszter Horanyi, Malinda Schaefer Zarske (This high school curriculum was originally created as a class project by engineering students in a Building Systems Program course at CU-Boulder.)Supporting Program
Integrated Teaching and Learning Program, College of Engineering and Applied Science, University of Colorado BoulderAcknowledgements
The contents of these digital library curricula were developed by the Integrated Teaching and Learning Program under National Science Foundation GK-12 grant no. 0338326. However, these contents do not necessarily represent the policies of the National Science Foundation, and you should not assume endorsement by the federal government.
Last modified: January 17, 2018
User Comments & Tips