Quick Look
Grade Level: 11 (11-12)
Time Required: 45 minutes
Lesson Dependency:
Subject Areas: Biology, Physical Science, Reasoning and Proof
NGSS Performance Expectations:
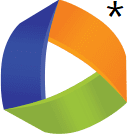
HS-PS2-6 |
Summary
Students are introduced to the concept of viscoelasticity and some of the material behaviors of viscoelastic materials, including strain rate dependence, stress relaxation, creep, hysteresis and preconditioning. Viscoelastic material behavior is compared to elastic solids and viscous fluids. Students learn about materials that have viscoelastic behavior along with the importance of engineers understanding viscoelasticity. To best engage the students, conduct the first half of the associated Creepy Silly Putty activity before conducting this lesson.Engineering Connection
When designing devices, engineers must understand how the materials that they select will react to the forces that the devices encounter. Since some materials are viscoelastic, engineers must know which materials these are and understand their behaviors. Bioengineers also study biological materials and how they function in healthy and diseased states. Biological materials are viscoelastic so bioengineers must understand viscoelastic behavior to fully characterize the behavior of these materials. As part of the process, engineers must also understand the environments in which their devices will operate to ensure effective and successful performance. For example, if designing a device to operate on viscoelastic materials in the human body, an engineer must understand and take into consideration how that environment will react to the forces imposed by the device.
Learning Objectives
After this lesson, students should be able to:
- Demonstrate an understanding of viscoelastic material behavior including strain rate dependence, stress relaxation, creep, hysteresis and preconditioning.
- Communicate scientific information about why the molecular-level structure of viscoelastic materials is important in the functioning of designed materials.
- List the types of materials that are viscoelastic.
Educational Standards
Each TeachEngineering lesson or activity is correlated to one or more K-12 science,
technology, engineering or math (STEM) educational standards.
All 100,000+ K-12 STEM standards covered in TeachEngineering are collected, maintained and packaged by the Achievement Standards Network (ASN),
a project of D2L (www.achievementstandards.org).
In the ASN, standards are hierarchically structured: first by source; e.g., by state; within source by type; e.g., science or mathematics;
within type by subtype, then by grade, etc.
Each TeachEngineering lesson or activity is correlated to one or more K-12 science, technology, engineering or math (STEM) educational standards.
All 100,000+ K-12 STEM standards covered in TeachEngineering are collected, maintained and packaged by the Achievement Standards Network (ASN), a project of D2L (www.achievementstandards.org).
In the ASN, standards are hierarchically structured: first by source; e.g., by state; within source by type; e.g., science or mathematics; within type by subtype, then by grade, etc.
NGSS: Next Generation Science Standards - Science
NGSS Performance Expectation | ||
---|---|---|
HS-PS2-6. Communicate scientific and technical information about why the molecular-level structure is important in the functioning of designed materials. (Grades 9 - 12) Do you agree with this alignment? |
||
Click to view other curriculum aligned to this Performance Expectation | ||
This lesson focuses on the following Three Dimensional Learning aspects of NGSS: | ||
Science & Engineering Practices | Disciplinary Core Ideas | Crosscutting Concepts |
Communicate scientific and technical information (e.g. about the process of development and the design and performance of a proposed process or system) in multiple formats (including orally, graphically, textually, and mathematically). Alignment agreement: | Attraction and repulsion between electric charges at the atomic scale explain the structure, properties, and transformations of matter, as well as the contact forces between material objects. Alignment agreement: | Investigating or designing new systems or structures requires a detailed examination of the properties of different materials, the structures of different components, and connections of components to reveal its function and/or solve a problem. Alignment agreement: |
International Technology and Engineering Educators Association - Technology
-
Explain how knowledge gained from other content areas affects the development of technological products and systems.
(Grades
6 -
8)
More Details
Do you agree with this alignment?
State Standards
Colorado - Science
-
Matter has definite structure that determines characteristic physical and chemical properties
(Grades
9 -
12)
More Details
Do you agree with this alignment?
Worksheets and Attachments
Visit [www.teachengineering.org/lessons/view/cub_surg_lesson04] to print or download.Pre-Req Knowledge
Understand the content in Mechanics of Elastic Solids and Viscous Fluids lessons, as well as an understanding of algebra, how to solve algebraic problems, and how to read and interpret graphs. Before starting this lesson, have students complete Part 1 of the Creepy Silly Putty activity, but not Part 2.
Introduction/Motivation
Note to teacher: We recommend that you conduct the first half of the associated Creepy Silly Putty activity before presenting students with the lesson content. This way, they think about the properties of solids and fluids and predict how they think silly putty behaves while they are able to touch it. This especially helps visual and kinesthetic learners to make their predictions. If you conduct the lesson first, then you give away the answer (that silly putty is viscoelastic). Thus, the recommended order to conduct the lesson and its two associated activities is as follows:
- Introduction/Motivation and Procedures-Part 1 (making and playing with silly putty) of the Creepy Silly Putty activity
- Viscoelasticity lesson
- Remainder of the Creepy Silly Putty activity (Procedures-Part 2:experimentation and testing)
- Preconditioning Balloons: Viscoelastic Biomedical Experiments activity
(In advance, make copies of the Viscoelasticity Worksheet, one per student, and have ready the silly putty that students created in the Creepy Silly Putty activity, or use purchased Silly Putty, to illustrate the principle of strain rate dependence. Also, as an option, prepare to show students the attached 10-slide PowerPoint Viscoelasticity Presentation to accompany the presented material. The slides are "animated" so you click the mouse (or space bar) to make the next item appear.)
What is a solid? What is a fluid? (Listen to student answers.) A solid is a material that has structural rigidity and resistance to change in shape or volume. In other words, solids maintain their shapes and do not form to their containers. A fluid is a material that flows to take the shape of its container. We have introduced how engineers calculate the material properties and behavior of solids and fluids.
Keeping in mind the definitions of a solid and a fluid, and the content we have learned so far, do you think silly putty behaves like an elastic solid or a viscous fluid? (Listen to student answers.) Both answers are correct! Silly putty behaves like an elastic solid and a viscous fluid depending on the forces that are applied to the material. If you put silly putty in a container, over time it deforms to take the container shape. However, if you stretch the silly putty fast, then it exhibits a well-defined failure that is similar to elastic solids. What do we call this? This is defined as viscoelastic material behavior.
Many materials have viscoelastic behavior. They are categorized into two different material groups: polymers and biological materials. On a molecular level, a polymer is defined as a material composed of a compound that is repeated to form a chain. Imagine that we are all the same compound. If we join hands then we form a long chain of the same compound connected together. This is how the compounds in a polymer are organized. The most common examples of polymers are plastics and rubbers. While many biological materials are also considered polymers because of their structures, they are defined as living materials. Both polymers and biological materials have viscoelastic behavior.
Why is it important to understand viscoelasticity? If engineers use viscoelastic materials in their designs or if their devices are intended to operate in environments with viscoelastic materials, it is imperative that they understand how viscoelastic materials behave. As an example, during the construction of the Fort Point Channel Tunnel in Boston, concrete ceiling panels were hung using bolts embedded in epoxy (a type of polymer). Over time, the bolts pulled out of the epoxy causing a three-ton panel to crash on the roadway below. The panel landed on a car carrying a young couple, killing the female passenger and injuring the male driver. The epoxy used was a viscoelastic material that deforms over time when a force is applied to it until it reaches an equilibrium state (creep). In this case, the concrete panel weighed too much for the epoxy and caused it to deform to the point of failure. The failure of this panel set off a chain reaction that eventually led to 12 tons of concrete falling to the roadway below (see Figure 1). After inspection of the tunnel, 242 unsafe bolts were identified. Had engineers fully understood viscoelasticity, this incident might have been avoided. You can see that it is important for all types of engineers to fully understand viscoelasticity if they are going to design devices that use or interact with polymers or biological materials.
(Continue on to present to students the content in the Lesson Background section.)
Lesson Background and Concepts for Teachers
(Present the following information to students, with or without the attached PowerPoint presentation.)
Viscoelasticity: Viscoelastic materials exhibit both viscous and elastic characteristics when undergoing deformation. This results in time-dependent behavior, which means that a material's response to deformation or force may change over time. Typical engineering materials have the same response to a force or deformation no matter how fast you apply the force/deformation or how long the force/deformation is present. Think of the Using Hooke's Law to Understand Materials activity: When you hang a weight on a spring it stretches to a certain displacement. If you take the weight off and put it back on it goes to the same displacement. If you let the weight hang from the spring for an hour, it does not change its displacement. If you slowly or quickly hang the weight on the spring, it still goes to the same displacement. This is not the case for viscoelastic materials; all of these factors can change how the material responds. The following material introduces some typical behaviors of viscoelastic materials.
Strain Rate Dependence: Viscoelastic materials respond differently depending on how fast they are stretched. Remember that displacement (or stretch) is related to strain, so the strain rate defines how fast the material is stretched. Therefore, viscoelastic materials are said to be strain rate-dependent. This can be demonstrated easily with silly putty. (optional: Have students use silly putty to illustrate this principle. Encourage students to stretch their silly putty at different rates and observe the behavior). If you slowly stretch silly putty (slow strain rate), the material seems to stretch forever, is very pliable, and behaves more like a highly viscous fluid. If you quickly stretch the silly putty (fast strain rate), then it breaks immediately without much displacement, seems stiff, and behaves more like a solid with little elasticity. The same material has two very different responses to a force depending on how fast the force was applied! It seems that faster strain rates cause more elastic responses and the material behaves more like a solid (stiffer), whereas slower strain rates cause more fluid-like behavior. This is a common characteristic of viscoelastic materials.
Figure 2 shows the difference in response of a biological material when it is stretched fast compared to slow. Both tests produced an initially curved response followed by a linear region, which is a typical stress-strain curve of viscoelastic materials. The fast strain rate produced a smaller curved region, in which the radius of curvature is small. The slow strain rate produced a very large curved region with a large radius of curvature. The linear region of the fast strain rate has a larger slope than the slow strain rate. This graph supports qualitative observations of silly putty. The curved region represents the viscous response of the material; a larger curved region suggests a more viscous response. The linear region represents the elastic response of the material; a larger slope represents a stiffer material.
Stress Relaxation: If you apply a constant displacement to a viscoelastic material, then the force to hold the material in this configuration decreases over time. An example of stress relaxation is when a rubber band, which is a polymer, is wrapped around a newspaper for an extended period of time. The rubber band is held at a constant displacement, however the force that it is applying to the newspaper decreases over time and it loses its integrity. The force continues to decrease until the material reaches an equilibrium in which the force becomes constant.
Figure 3 shows a stress vs. time graph of a material that was stretched to a certain displacement and held at that displacement over time. The initial vertical line represents the amount of force or stress it took to displace the material. As the material is held at that displacement, the stress in the material (or force it takes to hold the material at that displacement) decreases over time. Towards the end of the graph, the line becomes horizontal, indicating that the stress is no longer changing and the material has reached equilibrium.
Creep: If you apply a constant force to a viscoelastic material, then the displacement increases over time. When this force is released, it takes time for the material to recover to its initial configuration. An example of creep is when a bungee cord (a polymer) is used to hang a bike from the ceiling to save floor space. The bike is the constant force, so the bungee cord lengthens over time. Similar to stress relaxation, the displacement increases until the material reaches an equilibrium in which the displacement becomes constant. This was the cause of failure of the epoxy holding the concrete ceiling panels in the Fort Point Channel Tunnel (see Figure 1). The epoxy that held the bolts underwent creep and never reached equilibrium before the panels fell.
Figure 4 shows a strain vs. time graph of a material that had a force applied to it for a period of time and then removed. The initial vertical line represents the initial strain that the material experienced due to the force. This is similar to springs that immediately stretch when a force is applied. With the force constantly applied, the material continues to strain (or displace) over time. Eventually the material reaches equilibrium, which is seen by the horizontal line. When the force is removed, the initial decrease in strain is equal to the amount of strain the material instantaneously experienced when it first had the force applied. Over time, the material returns to its original configuration and its strain becomes zero.
Hysteresis: When a weight is applied to a spring, the spring stores energy to be able to return to its original configuration once the weight is removed. The amount of energy stored is equal to the amount of energy it took to displace the spring. When viscoelastic materials have a force applied to them and then removed, it takes more energy to displace the material than it does to return the material to its original configuration. In other words, it consumes more energy during the loading phase (applying a load and stretching the material) compared to the unloading phase (taking the load away and allowing the material to return to its original state). This energy difference is caused by the material losing energy during the loading phase, due to heat dissipation or molecular rearrangement within the material. Engineers calculate the amount of energy that was lost by analyzing the stress-strain diagram generated while stretching (loading) and unstretching (unloading) the material. The area between the loading and unloading curve represents the energy lost.
Figure 5 shows a stress-strain curve that was generated by loading and unloading a biological material. The loading portion is the top curve and the unloading region is the bottom curve. The area between the two lines is equal to the energy lost during this process.
Preconditioning: If the viscoelastic material is continued through this loading and unloading process, then the amount of energy lost in a cycle decreases until it reaches equilibrium close to zero energy lost. The amount of force it takes to displace the material also decreases with more cycles until the equilibrium point is reached. Exposing a viscoelastic material to this type of cyclic loading allows the viscous part of the material response to be dissipated and only the elastic portion remains. This is why the material is able to reach equilibrium. The viscous part of a material response can be difficult to fully characterize and understand, but the elastic behavior is easily understood and repeatable, making preconditioning useful to engineering researchers who need to compare elastic solids to viscoelastic materials. Many people also (usually unknowingly) take advantage of this unique characteristic of viscoelastic materials when blowing up balloons. It is common to see people using their hands to repeatedly stretch the balloon material before blowing them up. This preconditions the balloon with the purpose to reduce the amount of force required to stretch or inflate it. It is easier to blow up a balloon after preconditioning it.
Figure 6 shows a force vs. displacement graph of this response. The area between the lines of each cycle decreases and the peak force of each cycle decreases. Notice how large the difference is between the first and second cycle. This is the largest change observed between cycles. After the second cycle, the peak force and hysteresis continue to decrease but not as dramatically. The material eventually reaches equilibrium at which point it becomes hard to pick out the different cycles because they are overlapped.
Associated Activities
- Creepy Silly Putty - Towards achieving an understanding of how biological materials function, students learn about viscoelastic material behavior (strain rate dependence and creep) by making and testing different formulations of silly putty—a polymer material. It is recommended that students perform Part 1of the activity first, then receive the content and concept information in the Viscoelasticity lesson, and then complete Part 2 of the activity. Students collect data, make and interpret graphs, and material properties to elucidate material behavior.
- Preconditioning Balloons: Viscoelastic Biomedical Experiments - Students use balloons (a polymer) to explore preconditioning—a viscoelastic material behavior. They measure the force needed to stretch a balloon to the same displacement multiple times, and gain experience in data collection and graph interpretation.
Lesson Closure
We have learned that viscoelastic materials behave very differently than elastic materials. They exhibit time-dependent material behavior, such as strain rate dependence, stress relaxation, creep, hysteresis and preconditioning. What might change how viscoelastic materials respond to force? (Listen to student responses.) Yes, we learned that the rate at which a force is applied to a material, how long a force/deformation is applied to a material, and how many times that force is applied and removed can change how viscoelastic materials respond.
How does this information affect engineers? (Listen to student responses?) When designing devices, engineers must fully understand these properties if the materials they have selected or the environment that their devices will operate in is viscoelastic. If the devices are interacting with viscoelastic materials, then engineers must understand how those materials respond to forces so that failure can be prevented, and so that the devices perform successfully as intended.
Vocabulary/Definitions
elasticity: When a material instantaneously returns to its original shape after a force is removed.
equilibrium : A constant (steady) state of a material.
radius of curvature: The radius of a circle that would best fit a curved line.
viscoelasticity: When a material exhibits both viscous and elastic characteristics when undergoing deformation.
Assessment
Hands-On In Class: During the lesson, have students get out the silly putty that they made during the associated Creepy Silly Putty activity (or use purchased Silly Putty) and experiment with it:
- Encourage them to stretch the silly putty slowly until failure and compare its response to when it is stretched quickly until failure. This comparison demonstrates strain rate dependence.
- To observe creep, form the silly putty into a cylinder shape and hold the top of the cylinder with your hands so the silly putty deforms under its own weight. The weight of the silly putty is constant but the deformation continues to increase with time, which is the definition of creep.
- Have students note their observations of silly putty behavior and compare them to the spring behavior observed in the Using Hooke's Law to Understand Material activity.
Worksheet: After the lesson, have students complete the attached Viscoelasticity Worksheet. Review their answers to gauge their mastery of the subject matter.
Extension Activity: Research the "Big Dig" ceiling collapse online. Write a short paragraph describing what caused the failure and why it is important for engineers to understand viscoelasticity. Things to include:
- What caused the failure and why? Include the scientific terms you learned today, such as (but not limited to) viscoelasticity, deformation, polymer, and creep.
- Why is it important for engineers to understand viscoelasticity?
- Provide an explanation of an experiment that the engineers working on the Big Dig could have performed to avoid this catastrophe.
Example paragraph:
During the construction of the Fort Point Channel Tunnel in Boston, concrete ceiling panels were hung using bolts embedded in epoxy (a type of polymer). Over time, the bolts pulled out of the epoxy, causing a three-ton panel to crash on the roadway below. The bolts pulled out of the epoxy because viscoelastic materials deform over time when force applied to them until reaching equilibrium (creep). The panels weighed too much, which caused too large a deformation before reaching equilibrium. It is important to understand viscoelasticity so that you understand how the materials in your design will behave under certain conditions. This helps engineers to safely design devices and structures that do not fail and put people at risk. In this scenario, the engineers could have avoided this situation by testing various epoxy/polymer strength in a lap. They could determine which polymers deform over time and which don't to make an informed decision on which polymer would be appropriate for the design.
Additional Multimedia Support
Find more information about polymers at the University of Southern Mississippi's Polymer Science Learning Center (What are polymers and why do they act the way they do? Where are polymers? Types of polymers and how they are used. Making stuff with polymers. Polymer games and slimes.) at: http://pslc.ws/macrog/kidsmac/index.htm.
Learn more about the Boston tunnel incident at Wikipedia's Big Dig Ceiling Collapse website at http://en.wikipedia.org/wiki/Big_Dig_ceiling_collapse.
Subscribe
Get the inside scoop on all things TeachEngineering such as new site features, curriculum updates, video releases, and more by signing up for our newsletter!More Curriculum Like This

Students calculate stress, strain and modulus of elasticity, and learn about the typical engineering stress-strain diagram (graph) of an elastic material.

tudents are introduced to the similarities and differences in the behaviors of elastic solids and viscous fluids. In addition, fluid material properties such as viscosity are introduced, along with the methods that engineers use to determine those physical properties.

Students learn why engineers must understand tissue mechanics in order to design devices that will be implanted or used inside bodies, to study pathologies of tissues and how this alters tissue function, and to design prosthetics. Students learn about collagen, elastin and proteoglycans and their ro...

Students learn about viscoelastic material behavior, such as strain rate dependence and creep, by using silly putty, an easy-to-make polymer material. They learn how to make silly putty, observe its behavior with different strain rates, and then measure the creep time of different formulations of si...
Copyright
© 2011 by Regents of the University of Colorado.Contributors
Brandi N. Briggs; Marissa H. ForbesSupporting Program
Integrated Teaching and Learning Program, College of Engineering, University of Colorado BoulderAcknowledgements
This digital library content was developed by the Integrated Teaching and Learning Program under National Science Foundation GK-12 grant no. DGE 0338326. However, these contents do not necessarily represent the policies of the National Science Foundation, and you should not assume endorsement by the federal government.
Last modified: March 17, 2018
User Comments & Tips