Quick Look
Grade Level: 11 (11-12)
Time Required: 30 minutes
Lesson Dependency:
Subject Areas: Biology, Life Science
NGSS Performance Expectations:
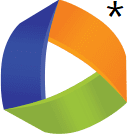
HS-PS2-6 |
Summary
Students reflect on their experiences making silly putty (the previous hands-on activity in the unit), especially why changing the borax concentration alters the mechanical properties of silly putty and how this pertains to tissue mechanics. Students learn why engineers must understand tissue mechanics in order to design devices that will be implanted or used inside bodies, to study pathologies of tissues and how this alters tissue function, and to design prosthetics. Finally, students learn about collagen, elastin and proteoglycans and their roles in giving body tissues their unique functions. This prepares them for the culminating design-build-test activity of the unit. > Image caption: Collagen is one example of a protein found in body tissues that alters the mechanical properties of the tissue to achieve its specific function.Engineering Connection
Engineers who design devices that will be implanted or used in human bodies must understand tissue mechanics to ensure that their devices restore function to injured or diseased tissues without harming surrounding tissues. Engineers also use tissue mechanics to study pathologies and their effect on tissue functionality. They use this knowledge to design devices that restore functionality to diseased tissues. Finally, engineers who design prosthetics use tissue mechanics to study the forces that biological arms, legs, hands or feet normally withstood and the previous range of motion, so as to design devices that restore those functions.
Learning Objectives
After this lesson, students should be able to:
- Explain, on a molecular level, how silly putty is formed and how this relates to tissue mechanics.
- Give three examples of why it is important for engineers to understand tissue mechanics.
- Describe the importance of collagen in human tissues and give an example of a tissue that uses collagen to function correctly.
- Describe the importance of elastin in human tissues and give an example of a tissue that uses elastin to function correctly.
- Describe the importance of proteoglycans in human tissues and give an example of a tissue that uses proteoglycans to function correctly.
Educational Standards
Each TeachEngineering lesson or activity is correlated to one or more K-12 science,
technology, engineering or math (STEM) educational standards.
All 100,000+ K-12 STEM standards covered in TeachEngineering are collected, maintained and packaged by the Achievement Standards Network (ASN),
a project of D2L (www.achievementstandards.org).
In the ASN, standards are hierarchically structured: first by source; e.g., by state; within source by type; e.g., science or mathematics;
within type by subtype, then by grade, etc.
Each TeachEngineering lesson or activity is correlated to one or more K-12 science, technology, engineering or math (STEM) educational standards.
All 100,000+ K-12 STEM standards covered in TeachEngineering are collected, maintained and packaged by the Achievement Standards Network (ASN), a project of D2L (www.achievementstandards.org).
In the ASN, standards are hierarchically structured: first by source; e.g., by state; within source by type; e.g., science or mathematics; within type by subtype, then by grade, etc.
NGSS: Next Generation Science Standards - Science
NGSS Performance Expectation | ||
---|---|---|
HS-PS2-6. Communicate scientific and technical information about why the molecular-level structure is important in the functioning of designed materials. (Grades 9 - 12) Do you agree with this alignment? |
||
Click to view other curriculum aligned to this Performance Expectation | ||
This lesson focuses on the following Three Dimensional Learning aspects of NGSS: | ||
Science & Engineering Practices | Disciplinary Core Ideas | Crosscutting Concepts |
Communicate scientific and technical information (e.g. about the process of development and the design and performance of a proposed process or system) in multiple formats (including orally, graphically, textually, and mathematically). Alignment agreement: | Attraction and repulsion between electric charges at the atomic scale explain the structure, properties, and transformations of matter, as well as the contact forces between material objects. Alignment agreement: | Investigating or designing new systems or structures requires a detailed examination of the properties of different materials, the structures of different components, and connections of components to reveal its function and/or solve a problem. Alignment agreement: |
International Technology and Engineering Educators Association - Technology
-
Medical technologies include prevention and rehabilitation, vaccines and pharmaceuticals, medical and surgical procedures, genetic engineering, and the systems within which health is protected and maintained.
(Grades
9 -
12)
More Details
Do you agree with this alignment?
State Standards
Colorado - Science
-
Develop, communicate, and justify an evidence-based scientific explanation supporting the current models of chemical bonding
(Grades
9 -
12)
More Details
Do you agree with this alignment?
Worksheets and Attachments
Visit [www.teachengineering.org/lessons/view/cub_surg_lesson05] to print or download.Pre-Req Knowledge
Students should understand the content presented in the Viscoelasticity lesson and have completed the Creepy Silly Putty activity.
Introduction/Motivation
(Optional: Use the Tissue Mechanics Presentation, a five-slide PowerPoint file, as you present students with the following lesson content.)
In our previous lesson, we learned about viscoelastic material behavior. These materials are not purely elastic or viscous, but a unique mixture of the two. The result is time-dependent material behavior. Two categories of materials are viscoelastic: polymers and biological materials. These materials have different responses to forces depending on the rate at which a force is applied, how long the force is applied, and how many times the force is applied and removed. To help us learn about viscoelastic behavior, we made three different concentrations of silly putty and observed their differences in behavior. What did we learn? We found that the more borax you add to the glue, the stiffer the silly putty and the more time it requires to creep a specified distance. What were your predictions of why you thought the concentration of borax altered the viscoelastic properties of silly putty? (Listen to student responses.)
Glue is a polymer, which means that it is made of long chains of molecules. When borax is added to glue it cross-links (that is, bonds) the long chains of molecules. Think of a plate of spaghetti. When you first drain the water from cooked spaghetti and put it on a plate, the spaghetti noodles are random and the individual noodles can easily slide over each other. This is equivalent to the long chains of molecules in the glue. As water evaporates from the spaghetti, the noodles start to stick together and become more of a solid mass. This is equivalent to what the borax does to the glue. It causes the molecule chains to stick to each other and to take on some elastic solid characteristics rather than just fluid behavior. Varying the amount of cross-links alters the material properties of the silly putty. Do you think more borax increases or decreases the stiffness of the silly putty? (Listen to student responses.) It increases the stiffness because the more borax present, the more cross-links that form!
So what does this have to do with tissue mechanics? The tissues in the human body contain varying degrees of collagen, a long protein strand (see Figure 1). Collagen can be compared to the long strands of molecules found in glue. Collagen can also cross-link in our bodies, similar to how the borax cross-links glue to form silly putty. Just as we demonstrated with varying the concentration of borax in our silly putty, the amount of collagen cross-linking in our tissues is directly related to the material properties of that tissue. As the concentration of collagen cross-links increases, so does tissue stiffness!
Lesson Background and Concepts for Teachers
Why study tissue mechanics?
Engineers must understand tissue mechanics if they design devices that will be implanted or used inside of the body. In order to make sure patients will not be harmed, they must know how their devices will affect surrounding tissues. The engineering challenge of designing implants, such as artificial heart valves (see Figure 2) and arterial stents, is to replace the functionality of natural tissues while not damaging surrounding tissues. Thus, engineers study the mechanics of the tissues that they are replacing, as well as how the surrounding tissues respond to the forces from their devices. Materials that are used in the body, such as surgical devices, must be biocompatible and not harm patients. Again, engineers must understand how tissues will respond to the forces from their devices to ensure correct function without negative side effects.
Engineers also use what they know about tissue mechanics to understand pathologies and their effect on tissues. For example, the disease of valve stenosis results in the stiffening of heart valves. Engineer can study exactly how the properties of the heart valve change with the disease to know how its function is affected. If it is determined that the tissue can no longer function correctly then engineer design a device to replace that function. Another pathology that engineers might study is osteoporosis (see Figure 3). This disease weakens the bones so they are more susceptible to breaking. In this case, engineers study how the disease affects the bone properties to determine if the diseased bone can function as it should. If the bone function is compromised, then engineers relay that knowledge to patients in order to help prevent future damage or injury. Sometimes engineers can understand what causes a disease by studying how a tissue has been altered and then working towards designing methods of prevention of that disease. Refer to the associated activity Designing a Robotic Surgical Device for students to act as engineers to design prototypes of laparoscopic surgical robots—remotely controlled, camera-toting devices that must fit through small incisions, inspect organs and tissue for disease, obtain biopsies, and monitor via ongoing wireless image-taking.
Finally, engineers use their knowledge of tissue mechanics to design prosthetics (see Figure 4), which are replacement body parts. Engineers must understand the forces that the biological (natural) arm, leg, hand or foot normally withstood, as well as the previous range of motion, in order to design replacement devices that restore function without fracturing the prosthesis when used.
What gives body tissues different properties?
We already discussed how body tissues contain collagen cross-links to alter the tissue stiffness, so what are other examples of components in our tissues that alter the mechanics? Let's discuss three examples: collagen, elastin and proteoglycans.
Collagen is a protein that is often present in the tissues of cartilage and tendons to provide strength. Collagen strands can be random in direction (like a plate of cooked spaghetti) to provide uniform properties in all directions or be aligned like the fibers in a rope to provide high-strength in one direction. An example of a tissue with aligned collagen is a tendon. Tendons connect muscles to bones and must be very strong in one direction so that they do not break when pulled on. The collagen in a tendon enable it to achieve this strength.
Elastin is a protein that provides tissues with elastic properties, like the springs we learned about in the Mechanics of Elastic Solids lesson and Using Hooke's Law to Understand Materials activity. An example of a tissue with a large concentration of elastin is an artery. The arteries in our bodies must be able to expand and contract to accommodate the varying pressure of our blood as it is pumped out of our heart. The elastin allows the artery to expand as the pressure increases and then quickly spring back to its original configuration helping the blood to move through the circulatory system. The largest pressures are experienced in the arteries closest to the heart; these arteries have the largest concentration of elastin.
Proteoglycans are proteins that attract water molecules, enabling tissues to retain water. This gives tissues more of the viscous fluid-like properties discussed in the Viscous Fluids lesson and Measuring Viscosity activity. Tissues that retain a large concentration of water are also used for lubrication throughout our bodies. An example of this type of tissue is cartilage. Cartilage is found in our joints to lubricate points where bone may come into contact with bone. When this cartilage becomes damaged and starts to deteriorate, we feel pain from our bones rubbing against each other during movement. Thus, te proteoglycans in the cartilage are imperative for performing daily activities pain free.
It is the unique combination of these components, in addition to other proteins, that gives the tissues in our bodies their specific properties so that they can achieve the correct and necessary functioning. Studying this combination, the role that each component plays, and how tissues changes with injury or disease provides engineers with the understanding to develop therapies to repair tissue and restore function.
Associated Activities
- Designing a Robotic Surgical Device - Student teams design prototypes of laparoscopic surgical robots—remotely controlled, camera-toting devices that must fit through small incisions, inspect organs and tissue for disease, obtain biopsies, and monitor via ongoing wireless image-taking. They use a synthetic abdominal cavity simulator to test and iterate the prototype devices.
Lesson Closure
Engineers must understand tissue mechanics in order to design devices that will be used in the body, to study pathologies and their effect on tissue properties, and to design prosthetics. Through understanding the healthy tissue's properties and functionality, engineers design devices that successfully restore function without harming surrounding tissues. Engineers also study how tissue properties, in combination with the protein composition, change with disease progression, which helps them to better understand the mechanisms of the disease so they can develop therapies to repair the tissue and restore function.
Vocabulary/Definitions
arterial stent: A tube placed in an artery to keep it open. Often made of plastic or metal mesh.
biocompatible: Being able to coexist with living tissues without causing harm. A material that is safe to use in the body.
collagen: A protein in body tissue that provides strength. Present in skin, bone, cartilage, tendons and teeth. Composed of strong, insoluble fibers that act as connective tissue.
creep: The response of a viscoelastic material when a constant force is applied. The displacement of the material increases over time until equilibrium is reached.
elasticity (elastic): When a material instantaneously returns to its original shape after a force is removed.
elastin: A protein that provides tissues with elastic properties.
mechanics: The study of how materials and systems react to forces and displacements.
pathology: The study and diagnosis of disease.
polymer: A material composed of a compound that is repeated to form a chain. Common examples are plastics and rubbers.
prosthetic: An artificial limb or a replacement body part.
proteoglycan: A protein that attracts water molecules, allowing tissues to retain water, giving them viscous, fluid-like properties.
viscoelasticity: A characteristic of materials that exhibit both viscous and elastic properties when undergoing deformation.
Assessment
Worksheet: At lesson end, have students complete the Tissue Mechanics Worksheet. Review their answers to gauge their mastery of the subject matter.
Subscribe
Get the inside scoop on all things TeachEngineering such as new site features, curriculum updates, video releases, and more by signing up for our newsletter!Copyright
© 2011 by Regents of the University of Colorado.Contributors
Brandi N. BriggsSupporting Program
Integrated Teaching and Learning Program, College of Engineering, University of Colorado BoulderAcknowledgements
This digital library content was developed by the Integrated Teaching and Learning Program under National Science Foundation GK-12 grant no. DGE 0338326. However, these contents do not necessarily represent the policies of the National Science Foundation, and you should not assume endorsement by the federal government.
Last modified: July 2, 2019
User Comments & Tips