Quick Look
Grade Level: 6 (6-7)
Time Required: 1 hour
Expendable Cost/Group: US $4.00 The activity uses mostly non-expendable (reusable) classroom and household supplies; see the Materials List for details.
Group Size: 28
Activity Dependency:
Subject Areas: Physical Science, Physics, Science and Technology
NGSS Performance Expectations:
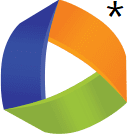
MS-PS3-1 |
MS-PS3-5 |
Summary
As a weighted plastic egg is dropped into a tub of flour, students see the effect that different heights and masses of the same object have on the overall energy of that object while observing a classic example of potential (stored) energy transferred to kinetic energy (motion). The plastic egg's mass is altered by adding pennies inside it. Because the egg's shape remains constant, and only the mass and height are varied, students can directly visualize how these factors influence the amounts of energy that the eggs carry for each experiment, verified by measurement of the resulting impact craters. Students review the equations for kinetic and potential energy and then make predictions about the depths of the resulting craters for drops of different masses and heights. They collect and graph their data, comparing it to their predictions, and verifying the relationships described by the equations. This classroom demonstration is also suitable as a small group activity.Engineering Connection
This activity models a real-world situation—asteroids hitting the moon's surface—providing students with an example of how engineers and other researchers often employ simple models to help predict how systems behave. By gaining exposure to the fundamental energy equations and initial experimental data, students are able to predict the expected outcome of different egg-drops and compare to experimental results. They learn what engineers know, that energy is influenced by the mass of an object and the height from which that object is dropped. Engineers use their understanding of the energy equations as they create new products, tools, systems and structures that are safe, robust and reliable.
Learning Objectives
After this activity, students should be able to:
- Predict how the potential energy of a system will change if the height of an object changes.
- Predict how the potential energy of a system will change if the mass of an object changes.
- Predict how the kinetic energy of a system will change if the velocity of an object changes.
Educational Standards
Each TeachEngineering lesson or activity is correlated to one or more K-12 science,
technology, engineering or math (STEM) educational standards.
All 100,000+ K-12 STEM standards covered in TeachEngineering are collected, maintained and packaged by the Achievement Standards Network (ASN),
a project of D2L (www.achievementstandards.org).
In the ASN, standards are hierarchically structured: first by source; e.g., by state; within source by type; e.g., science or mathematics;
within type by subtype, then by grade, etc.
Each TeachEngineering lesson or activity is correlated to one or more K-12 science, technology, engineering or math (STEM) educational standards.
All 100,000+ K-12 STEM standards covered in TeachEngineering are collected, maintained and packaged by the Achievement Standards Network (ASN), a project of D2L (www.achievementstandards.org).
In the ASN, standards are hierarchically structured: first by source; e.g., by state; within source by type; e.g., science or mathematics; within type by subtype, then by grade, etc.
NGSS: Next Generation Science Standards - Science
NGSS Performance Expectation | ||
---|---|---|
MS-PS3-1. Construct and interpret graphical displays of data to describe the relationships of kinetic energy to the mass of an object and to the speed of an object. (Grades 6 - 8) Do you agree with this alignment? |
||
Click to view other curriculum aligned to this Performance Expectation | ||
This activity focuses on the following Three Dimensional Learning aspects of NGSS: | ||
Science & Engineering Practices | Disciplinary Core Ideas | Crosscutting Concepts |
Construct and interpret graphical displays of data to identify linear and nonlinear relationships. Alignment agreement: Collect data to produce data to serve as the basis for evidence to answer scientific questions or test design solutions under a range of conditions.Alignment agreement: Use mathematical representations to describe and/or support scientific conclusions and design solutions.Alignment agreement: | Motion energy is properly called kinetic energy; it is proportional to the mass of the moving object and grows with the square of its speed. Alignment agreement: | Proportional relationships (e.g. speed as the ratio of distance traveled to time taken) among different types of quantities provide information about the magnitude of properties and processes. Alignment agreement: |
NGSS Performance Expectation | ||
---|---|---|
MS-PS3-5. Construct, use, and present arguments to support the claim that when the kinetic energy of an object changes, energy is transferred to or from the object. (Grades 6 - 8) Do you agree with this alignment? |
||
Click to view other curriculum aligned to this Performance Expectation | ||
This activity focuses on the following Three Dimensional Learning aspects of NGSS: | ||
Science & Engineering Practices | Disciplinary Core Ideas | Crosscutting Concepts |
Science knowledge is based upon logical and conceptual connections between evidence and explanations. Alignment agreement: | When the motion energy of an object changes, there is inevitably some other change in energy at the same time. Alignment agreement: | Energy may take different forms (e.g. energy in fields, thermal energy, energy of motion). Alignment agreement: |
Common Core State Standards - Math
-
Reason abstractly and quantitatively.
(Grades
K -
12)
More Details
Do you agree with this alignment?
-
Model with mathematics.
(Grades
K -
12)
More Details
Do you agree with this alignment?
-
Understand the concept of a ratio and use ratio language to describe a ratio relationship between two quantities.
(Grade
6)
More Details
Do you agree with this alignment?
-
Recognize and represent proportional relationships between quantities.
(Grade
7)
More Details
Do you agree with this alignment?
-
Construct and interpret scatter plots for bivariate measurement data to investigate patterns of association between two quantities. Describe patterns such as clustering, outliers, positive or negative association, linear association, and nonlinear association.
(Grade
8)
More Details
Do you agree with this alignment?
International Technology and Engineering Educators Association - Technology
-
Energy is the capacity to do work.
(Grades
6 -
8)
More Details
Do you agree with this alignment?
State Standards
California - Math
-
Reason abstractly and quantitatively.
(Grades
K -
12)
More Details
Do you agree with this alignment?
-
Model with mathematics.
(Grades
K -
12)
More Details
Do you agree with this alignment?
-
Understand the concept of a ratio and use ratio language to describe a ratio relationship between two quantities.
(Grade
6)
More Details
Do you agree with this alignment?
-
Recognize and represent proportional relationships between quantities.
(Grade
7)
More Details
Do you agree with this alignment?
-
Construct and interpret scatter plots for bivariate measurement data to investigate patterns of association between two quantities. Describe patterns such as clustering, outliers, positive or negative association, linear association, and nonlinear association.
(Grade
8)
More Details
Do you agree with this alignment?
California - Science
-
Construct, use, and present arguments to support the claim that when the kinetic energy of an object changes, energy is transferred to or from the object.
(Grades
6 -
8)
More Details
Do you agree with this alignment?
-
Construct and interpret graphical displays of data to describe the relationships of kinetic energy to the mass of an object and to the speed of an object.
(Grades
6 -
8)
More Details
Do you agree with this alignment?
Materials List
For the class demonstration (or each group):
- large square plastic container or small roasting tray, at least 12 x 12 x 5 inches (or more) deep
- flour to fill the container or tray to a depth of at least 4 inches (~$4 for a 5-pound bag)
- plastic Easter egg shell, such as at Party City
- 10 pennies (each penny is 2.4 g), for weight inside the plastic egg
- short ruler, 12 inches or less, including metric marks
- notecard
- materials to lay under/around the flour tray for easier cleanup,, such as a tarp, ground cloth, newspapers, butcher paper or large plastic bags
- yardstick (or meter stick)
- pile of books, bricks, or something similarly heavy, to prop up the yardstick near the flour tray
- Moon Craters Worksheet, one per student
Materials for optional activity:
- smart phone, one that you are okay dropping to the floor from a height of 2 feet
- small foam pad or padded surface on which the smart phone can fall, to reduce the risk of breaking it
- download Freefall Highscore, a smartphone app
For the entire class to share:
- graph paper and pencil, for each student
- short rulers, for graphing
- capability to project in the classroom a PowerPoint® file, Moon Crater Quizzes & Answers
- (optional) capability to show the class an online video
Worksheets and Attachments
Visit [www.teachengineering.org/activities/view/ucd_energy_lesson02_activity1] to print or download.Introduction/Motivation
Today we are going to make "moon craters." How do you think real moon craters are made? (Listen to a few student ideas.) Moon craters are caused by the impact of large, heavy objects moving at high speeds. These objects are called asteroids.
What determines how large the crater is? (Listen to student responses.) Researchers have learned that the more energy an asteroid has when it strikes the moon surface, the larger the resulting crater. (Show students the first question in the Moon Crater Quizzes & Answers, slide 2, to get them thinking about the mass and height of objects.) Which object do you think has more energy? (Go through the three pre-quizzes to gauge the level of students' intuition about energy as it relates to the mass and height of objects without giving away the answers.)
What else might influence the size of the craters made on the moon? (Listen to student ideas to see if anyone suggests "speed.")
Well we are going to test it in action to see what factors increase or decrease the amount of energy asteroids have! Who would like to volunteer to make the first moon crater?
Procedure
Background
Conduct the activity either as a class demonstration in which students volunteer and you guide them in the process, or in smaller groups. The activity may take longer than 60 minutes if done in smaller groups. Also, you may want to take 30 more minutes to better cover kinetic energy topics.
The impact crater that an egg leaves in the flour scales linearly at small masses and heights. Or put simply, the depth of the crater doubles if the mass or the height that the egg is dropped from doubles. By first showing students the equations for kinetic energy (KE = ½ x m x v2) and potential energy (PE = m x g x h), students are able to make predictions about what they think may happen to the depth of the impact crater given different masses and heights (the object's shape and speed, gravitational energy, remain constant).
Have students collect experimental data for drop tests at two different masses and three different heights. After two data points are collected, have students model or predict the remaining results and check their predictions against the experimental data. Note: As the mass and height of the egg increases beyond 24 grams or 2 feet, respectively, the crater depth becomes an increasingly poor approximation for the amount of energy.
Before the Activity
- Gather materials and make copies of the Moon Craters Worksheet.
- Practice the activity so you are aware of the setup, takedown and amount of mess to expect.
- Set up the demonstration in an area with enough space for students to gather around and be able to see the egg drops and landings. Use a pile of heavy books or bricks to position and securely prop up a vertical yardstick next to the flour tray, so the bottom of the yardstick is aligned with the top surface of the flour. Prepare the plastic egg shell with 12 g of weight (5 pennies; each penny weighs 2.4 g); when you are ready for the 24-g egg, add 5 more pennies (10 pennies total).
- If you are conducting the optional activity with the dropped cell phone, make a table setup to record the height and time it takes to fall a given distance. Download the Freefall Highscore app and test.
- Be ready to project in the classroom the Moon Crater Quizzes & Answers PowerPoint® file.
With the Students
- Present the Introduction/Motivation content, including the pre-quizzes. Review potential energy and kinetic energy with the class as presented in the Exploring Energy: Kinetic and Potential lesson. Write the energy equations on the board and discuss what each term represents. PE = m x g x h = potential energy is the product of mass, acceleration due to gravity, and height KE = ½ x m x v2 = kinetic energy is the product of one-half mass, and the square of velocity.
- Tell the students: This activity is about modeling moon craters, and using models to predict the size of moon craters. A model is a representation of something, sometimes on a different scale. (Do any of you have model cars, airplanes or railroads?) Models help us learn about something that cannot be directly observed or experimented on. Engineers often employ simple models like this to help them predict how systems behave and what might happen under certain conditions. Engineers also use the energy equations to make predictions about the amount of energy involved in a system because they are concerned with the effect of energy as it applies to all the inventions, devices and structures they design.
- Have students make predictions on their worksheets about what factors may increase the crater size. Expect them to guess factors such as force, velocity, size, shape and mass. Redirect their attention to the energy equations just presented. See if they guess mass, velocity, height and gravity. Let them know that they will be testing the variables mass and height on the impact of the crater.
- Experimental procedure for class demonstration: Ask for volunteers (or choose students) to perform each test or set of tests in the experiment, as outlined on the worksheet. Follow these basic steps:
- Use a notecard to smooth the flour surface so it is fairly level, with no obvious craters.
- Have a student hold an egg up to the specified height being tested and then drop the egg.
- Use a short ruler to measure the depth of the impact crater at its deepest point. Use the notecard to help you sight the surface height to the ruler.
- Have students record in their worksheet data tables the three replicate depth measurements (in cm or mm) for the different heights and masses that are tested.
- Next, direct students to graph their data, as instructed on the worksheet (and described in the Assessment section).
- If conducting the optional activity with the smartphone, follow the instructions below.
- Once data collection and graphing are completed, review the graphs as a class to guide an analysis of what conclusions can be drawn from looking at the data visualization.
- Conclude with a class discussion to reflect on the real-world implications from what students learned in the activity. See the Assessment section for suggested questions.
Optional Activity
- Using a smartphone and the Freefall Highscore app, record the time it takes for the phone to fall from each height that you tested during the crater experiment.
- Repeat each height 3 to 4 times, so that you have enough data to average the times together.
- If necessary, show students the 47-second YouTube video of a hammer and a feather dropping on the moon to convince them that the phone falls at the same velocity as the egg.
- Have students record in their worksheet data tables the lengths of time that the phone is in free fall.
- Next, direct students to use their data to create height vs. time and height vs. average velocity graphs (which requires them to calculate average velocity).
Vocabulary/Definitions
energy: The ability to make things happen. More advanced definition: The ability to do work.
energy conversion: The change of energy from one form to another.
kinetic energy: The energy of moving objects. Anything in motion has kinetic energy. The faster an object moves, the more kinetic energy it has.
model: (noun) A representation of something for imitation, comparison or analysis, sometimes on a different scale. (verb) To make something to help learn about something else that cannot be directly observed or experimented upon.
potential energy: Energy that is stored and can be used when needed. Energy can be stored in chemicals (food, batteries), height (gravitational), elastic stretching, etc.
Assessment
Pre-Activity Assessment
Pre-Quizzes: Prior to conducting the activity, take five minutes to administer the three-questions in the Moon Crater Quizzes & Answers PowerPoint® file, to determine what students already know about potential energy, kinetic energy and the factors that influence the amount of energy (mass, velocity and height from which an object falls). Review their answers to gauge their depth of base knowledge about these topics.
Activity Embedded Assessment
Predictions: As data is being collected, quiz students to see if they can predict the resulting crater depth. Using the energy equation PE = m x g x h, expect students to be able to predict that if the height is doubled (1 ft to 2 ft), the impact depth will double. Similarly, expect them to be able to predict that if the mass is doubled (12 g to 24 g), the impact depth will double. Record student predictions, and compare them to the measured experiment results after each drop.
Post-Activity Assessment
Graphing & Conclusions: After students have collected data, assign them to make height vs. impact depth graphs in the form of scatter plots or bar graphs, depending on the math lessons they are concurrently learning. Either use height and weight averages, or graph each trial independently. If the optional activity is conducted, also require height vs. time and height vs. average velocity graphs (which requires students to learn how to calculate average velocity). As a class, review the graphs to guide an analysis of what conclusions can be drawn from looking at the data visualization.
Engineering Reflection: In a concluding class discussion, reflect upon the real-world implications from what students observed and learned in the activity. Ask the students:
- Who might want to conduct an experiment like we did today—an experiment that models a real-world situation? Why? (Answer: Engineers do this to test for the consequences or results that might occur with the use of their inventions and designs. Engineers and other researchers often employ simple models like this to help them predict how systems behave and what might happen under different conditions. Scientists also do this to test their hypotheses about what might have happened to explain found physical evidence.)
- Who might want to use the kinetic energy and potential energy equations we learned today? (Answer: Engineers use these equations to make predictions about the amount of energy involved in a system because they are concerned with the effect of energy as it applies to all the inventions, products and structures they design.)
- When might engineers care about the effects of energy transfer and the factors of mass, velocity and height? (Answer: When engineers design something that involves energy and movement [lots of things!], they want it to work as intended and be safe, so they do "mock-up" tests to see what could happen and use what they learn to adjust the design solution to make it better.)
Safety Issues
The smartphone screen may break, but by taking the precaution to pad the surface on which it is dropped, it is exceptionally unlikely to break.
Troubleshooting Tips
Expect a scattering of flour during the experiment, so cover the area under and around the tray with newspapers (or other material) to simplify cleanup. If this activity is done as a class demonstration with a few student volunteers, cleanup is less of a problem than if students do the same activity in small groups throughout the classroom.
If students are unfamiliar with mathematical equations, graphing and interpreting figures, spend some time going over these topics before and after this activity to ground students in the basics.
Activity Extensions
Assign students to write about what factors might influence crater depths, and what sorts of asteroid characteristics they might expect to associate with bigger or smaller moon craters. As necessary, include a discussion to clarify that energy relates to the square of velocity (doubling velocity quadruples the amount of energy).
Subscribe
Get the inside scoop on all things TeachEngineering such as new site features, curriculum updates, video releases, and more by signing up for our newsletter!Copyright
© 2014 by Regents of the University of Colorado; original © 2013 University of California DavisContributors
Eric Anderson, Jeff Kessler, Irene ZhaoSupporting Program
RESOURCE GK-12 Program, College of Engineering, University of California DavisAcknowledgements
The contents of this digital library curriculum were developed by the Renewable Energy Systems Opportunity for Unified Research Collaboration and Education (RESOURCE) project in the College of Engineering under National Science Foundation GK-12 grant no. DGE 0948021. However, these contents do not necessarily represent the policies of the National Science Foundation, and you should not assume endorsement by the federal government.
Last modified: May 5, 2021
User Comments & Tips