Quick Look
Grade Level: 3 (3-5)
Time Required: 45 minutes
Expendable Cost/Group: US $1.00
Group Size: 1
Activity Dependency: None
Subject Areas: Earth and Space
NGSS Performance Expectations:
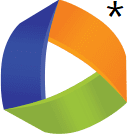
3-5-ETS1-1 |
3-5-ETS1-2 |
3-5-ETS1-3 |
3-ESS3-1 |
3-PS2-2 |
Summary
Students make sense of the design challenges engineers face that arise from earthquake phenomena. Students work as engineering teams to explore concepts of how engineers design and construct buildings to withstand earthquake damage by applying elements of the engineering design process by building their own model structures using toothpicks and marshmallows. The groups design, build, and test their model buildings and then determine how earthquake-proof their designs are by testing them on an earthquake simulator pan of Jell-O®.Engineering Connection
Because earthquakes can cause walls to crack, foundations to move and even entire buildings to crumple, engineers incorporate into their structural designs techniques that withstand damage from earthquake forces, for example, cross bracing, large bases and tapered geometry. Earthquake-proof buildings are intended to bend and sway with the motion of earthquakes, or are isolated from the movement by sliders. Engineers use the engineering design process to come up with an idea, test it, and then re-engineer the structure based on its performance.
Learning Objectives
After this activity, students should be able to:
- Identify some of the factors that make buildings earthquake-proof, including cross bracing, large "footprints," and tapered geometry.
- Model an earthquake-proof structure using simple materials.
- Compare a model structure with what it represents.
- Understand why engineers need to learn about earthquakes.
- Learn what causes earthquakes and how engineers use this knowledge to design more 'earthquake-proof' structures.
Educational Standards
Each TeachEngineering lesson or activity is correlated to one or more K-12 science,
technology, engineering or math (STEM) educational standards.
All 100,000+ K-12 STEM standards covered in TeachEngineering are collected, maintained and packaged by the Achievement Standards Network (ASN),
a project of D2L (www.achievementstandards.org).
In the ASN, standards are hierarchically structured: first by source; e.g., by state; within source by type; e.g., science or mathematics;
within type by subtype, then by grade, etc.
Each TeachEngineering lesson or activity is correlated to one or more K-12 science, technology, engineering or math (STEM) educational standards.
All 100,000+ K-12 STEM standards covered in TeachEngineering are collected, maintained and packaged by the Achievement Standards Network (ASN), a project of D2L (www.achievementstandards.org).
In the ASN, standards are hierarchically structured: first by source; e.g., by state; within source by type; e.g., science or mathematics; within type by subtype, then by grade, etc.
NGSS: Next Generation Science Standards - Science
NGSS Performance Expectation | ||
---|---|---|
3-5-ETS1-1. Define a simple design problem reflecting a need or a want that includes specified criteria for success and constraints on materials, time, or cost. (Grades 3 - 5) Do you agree with this alignment? |
||
Click to view other curriculum aligned to this Performance Expectation | ||
This activity focuses on the following Three Dimensional Learning aspects of NGSS: | ||
Science & Engineering Practices | Disciplinary Core Ideas | Crosscutting Concepts |
Define a simple design problem that can be solved through the development of an object, tool, process, or system and includes several criteria for success and constraints on materials, time, or cost. Alignment agreement: | Possible solutions to a problem are limited by available materials and resources (constraints). The success of a designed solution is determined by considering the desired features of a solution (criteria). Different proposals for solutions can be compared on the basis of how well each one meets the specified criteria for success or how well each takes the constraints into account. Alignment agreement: | People's needs and wants change over time, as do their demands for new and improved technologies. Alignment agreement: |
NGSS Performance Expectation | ||
---|---|---|
3-5-ETS1-2. Generate and compare multiple possible solutions to a problem based on how well each is likely to meet the criteria and constraints of the problem. (Grades 3 - 5) Do you agree with this alignment? |
||
Click to view other curriculum aligned to this Performance Expectation | ||
This activity focuses on the following Three Dimensional Learning aspects of NGSS: | ||
Science & Engineering Practices | Disciplinary Core Ideas | Crosscutting Concepts |
Generate and compare multiple solutions to a problem based on how well they meet the criteria and constraints of the design problem. Alignment agreement: | Research on a problem should be carried out before beginning to design a solution. Testing a solution involves investigating how well it performs under a range of likely conditions. Alignment agreement: At whatever stage, communicating with peers about proposed solutions is an important part of the design process, and shared ideas can lead to improved designs.Alignment agreement: | Engineers improve existing technologies or develop new ones to increase their benefits, to decrease known risks, and to meet societal demands. Alignment agreement: |
NGSS Performance Expectation | ||
---|---|---|
3-5-ETS1-3. Plan and carry out fair tests in which variables are controlled and failure points are considered to identify aspects of a model or prototype that can be improved. (Grades 3 - 5) Do you agree with this alignment? |
||
Click to view other curriculum aligned to this Performance Expectation | ||
This activity focuses on the following Three Dimensional Learning aspects of NGSS: | ||
Science & Engineering Practices | Disciplinary Core Ideas | Crosscutting Concepts |
Plan and conduct an investigation collaboratively to produce data to serve as the basis for evidence, using fair tests in which variables are controlled and the number of trials considered. Alignment agreement: | Tests are often designed to identify failure points or difficulties, which suggest the elements of the design that need to be improved. Alignment agreement: Different solutions need to be tested in order to determine which of them best solves the problem, given the criteria and the constraints.Alignment agreement: |
NGSS Performance Expectation | ||
---|---|---|
3-ESS3-1. Make a claim about the merit of a design solution that reduces the impacts of a weather-related hazard. (Grade 3) Do you agree with this alignment? |
||
Click to view other curriculum aligned to this Performance Expectation | ||
This activity focuses on the following Three Dimensional Learning aspects of NGSS: | ||
Science & Engineering Practices | Disciplinary Core Ideas | Crosscutting Concepts |
Make a claim about the merit of a solution to a problem by citing relevant evidence about how it meets the criteria and constraints of the problem. Alignment agreement: | A variety of natural hazards result from natural processes. Humans cannot eliminate natural hazards but can take steps to reduce their impacts. Alignment agreement: | Cause and effect relationships are routinely identified, tested, and used to explain change. Alignment agreement: Engineers improve existing technologies or develop new ones to increase their benefits (e.g., better artificial limbs), decrease known risks (e.g., seatbelts in cars), and meet societal demands (e.g., cell phones).Alignment agreement: Science affects everyday life.Alignment agreement: |
NGSS Performance Expectation | ||
---|---|---|
3-PS2-2. Make observations and/or measurements of an object's motion to provide evidence that a pattern can be used to predict future motion. (Grade 3) Do you agree with this alignment? |
||
Click to view other curriculum aligned to this Performance Expectation | ||
This activity focuses on the following Three Dimensional Learning aspects of NGSS: | ||
Science & Engineering Practices | Disciplinary Core Ideas | Crosscutting Concepts |
Make observations and/or measurements to produce data to serve as the basis for evidence for an explanation of a phenomenon or test a design solution. Alignment agreement: Science findings are based on recognizing patterns.Alignment agreement: | The patterns of an object's motion in various situations can be observed and measured; when that past motion exhibits a regular pattern, future motion can be predicted from it. (Boundary: Technical terms, such as magnitude, velocity, momentum, and vector quantity, are not introduced at this level, but the concept that some quantities need both size and direction to be described is developed.) Alignment agreement: | Patterns of change can be used to make predictions. Alignment agreement: |
Common Core State Standards - Math
-
Represent and solve problems involving multiplication and division.
(Grade
3)
More Details
Do you agree with this alignment?
-
Multiply and divide within 100.
(Grade
3)
More Details
Do you agree with this alignment?
-
Apply the formulas V = l × w × h and V = b × h for rectangular prisms to find volumes of right rectangular prisms with whole-number edge lengths in the context of solving real world and mathematical problems.
(Grade
5)
More Details
Do you agree with this alignment?
International Technology and Engineering Educators Association - Technology
-
Students will develop an understanding of the attributes of design.
(Grades
K -
12)
More Details
Do you agree with this alignment?
-
Students will develop an understanding of engineering design.
(Grades
K -
12)
More Details
Do you agree with this alignment?
-
Students will develop abilities to apply the design process.
(Grades
K -
12)
More Details
Do you agree with this alignment?
-
Models are used to communicate and test design ideas and processes.
(Grades
3 -
5)
More Details
Do you agree with this alignment?
-
Test and evaluate the solutions for the design problem.
(Grades
3 -
5)
More Details
Do you agree with this alignment?
State Standards
Colorado - Math
-
Multiply and divide within 100.
(Grade
3)
More Details
Do you agree with this alignment?
-
Represent and solve problems involving multiplication and division.
(Grade
3)
More Details
Do you agree with this alignment?
-
Apply the formulas V = l × w × h and V = b × h for rectangular prisms to find volumes of right rectangular prisms with whole-number edge lengths.
(Grade
5)
More Details
Do you agree with this alignment?
Materials List
Each student needs:
- 30 toothpicks
- 30 miniature marshmallows
- Making Sense Assessment
For the entire class to share:
- 8 square baking dishes, 21.5 cm x 21.5 cm (8 ½ inch x 8 ½ inch) or 1 oblong baking dish, 21.5 cm x 28 cm (8 ½ in x 11 inch)
- 8 boxes Jell-O, prepared according to package directions
Worksheets and Attachments
Visit [www.teachengineering.org/activities/view/cub_natdis_lesson03_activity1] to print or download.Introduction/Motivation
Show students parts of the following video of the ground moving during an earthquake (start at minute 1:25): https://www.youtube.com/watch?v=q5nf6Wl5TVI.
Tell students to draw a T-Chart in their notebooks. Label the first column “What I Notice” and the second column “What I Wonder”. Have students write down what they notice (observations) and wonder (questions) about the ground motion.
Ask students to share their observations and the questions they have.
Now show students the following video of various skyscrapers moving during an earthquake: https://www.youtube.com/watch?v=iGtRko8y4Fo.
Have students use their T-Charts to write down what they notice and wonder about the skyscrapers’ motion. Ask students to make sense of the motion and if they can detect a pattern. Ask students, “Is the reason the ground moves (seen in the first video) the same reason that is making the buildings move in this video? What is causing this motion?” Allow students to share their ideas and compile a list of ideas and common themes.
Earthquakes are a natural hazard that results in the very sudden and forceful shaking of the Earth’s crust. Earthquakes happen frequently at the convergence of tectonic plates. When these plates hit or slide past each other, they release built up stress. The surface where the tectonic plates slip is called a fault. The point where an earthquake occurs is called the focus of the earthquake, which can be close to or far below the Earth's surface.
The location on the Earth’s surface that is directly above the focus is called the epicenter of the earthquake. The epicenter is where most damage occurs. The release of force at the earthquake's focus creates vibrations that travel in seismic waves away from the epicenter. Earthquakes can crack walls and move foundations and may cause entire buildings to crumble. Large earthquakes can even cause loss of life and millions of dollars’ worth of damage. (Note: USGS’s “Earthquakes for Kids” offers additional information)
To keep people inside and outside of building safe in areas of high earthquake risk, civil engineers strive to design buildings that are more resilient to the damaging forces of earthquakes.
Ask students, “How do you think engineers design earthquake-proof buildings?”
Ask a few students to share their ideas, and then briefly introduce the engineering design process.
Explain that engineers ask critical questions about what they want to create or what specific problem they are trying to solve. These questions include: What are the project requirements (criteria)? What are the limitations (constraints)? This also requires conducting research on the problem. For example, civil engineers work together with scientists, as well as engineers from different backgrounds, to better understand the problem of how to design earthquake-proof buildings.
The engineering team then imagines possible solutions to the problem. This takes a lot of creative brainstorming! After making a list of solutions and some sketches of their ideas, the team of engineers plan and select their best idea.
Once the team decides on the details of the final design, they create the prototype and test it out! Prototypes are smaller models that are used by engineers to test a design. In the testing phase, the team pays attention to what needs to be changed to the model to make the product work better. The last step of the engineering design process is to improve (iterate) their design.
Procedure
Before the Activity
- Prepare the Jell-O the night before the activity so that it is fully set when students begin the activity. Pour the Jell-O into eight 21.5 cm (8½-inch) square pans to be shared by four students, or in one large pan for the entire class to share.
- Gather materials.
With the Students
- Explain to students that they will play the role of civil engineers and take on an engineering design challenge. Their challenge is to design and build models of earthquake-proof buildings, and then test their models to assess how well their structures stand up to ground motion during a simulated earthquake.
- Explain that engineers creatively work within constraints, such as limited resources, materials, time, or money. For this engineering design challenge, students are limited to using only mini marshmallows and toothpicks to build their model structures. In addition to the material constraints, students must also meet each of the following design constraints:
- Buildings must be at least 2 toothpick levels high
- Buildings must contain at least 1 triangle
- Buildings must contain at least 1 square
- Before starting to build their models, have students brainstorm and draw sketches of their building designs in their notebooks. For background knowledge, explain that cubes and triangles are like building blocks that may be stacked in different ways to make towers, and that buildings can have small or large "footprints" (bases). Have students draw and label the shapes in their designs (cube, triangle, etc.).
- Once students have drawn and labelled their sketches, distribute 30 toothpicks and 30 marshmallows to each student or team, and have them build their first model prototype.
- Before testing their models, show students the pan of Jell-O (without a structure on top) and tell them this represents the ground. Shake the pan back and forth in a shearing motion to simulate an earthquake. For fair testing, place two marks on the table indicating the distance the pans can be shaken with an even force and speed over a set a time for the duration of the “earthquake”.
- Next, have students test their structures one at a time on the Jell-O pan earthquake simulator. Shake the pan for the set time, force and distance.
- After the earthquake is over, have students make a quick sketch of their model building before removing it from the pan of Jell-O.
- As a class, have students compare and contrast their before-and-after sketches. Ask students to share and reflect on what worked and what did not work for their first model prototypes. Explain that engineers learn from “failure” and that this is essential information that helps them to design even better products and solutions. Ask students:
- Did their model structure stay the same, break, or fall?
- What ideas do students have to make their structure stronger?
- Which variable(s) would students change in their building design?
- Now that students have gone through their first design, build, and test cycle, show students the following photographs of different earthquake-proof buildings: https://www.frontiersin.org/files/Articles/272020/fbuil-03-00049-HTML/image_m/fbuil-03-00049-g005.jpg
Ask students:
- What do you notice about the design of the structures?
- What similarities do you see in building designs?
- What features do you think help make the buildings earthquake-proof?
Have students share their observations. Help students notice that the structures have a large base (smaller at the top) and a cross-bracing design.
Follow-up with asking students:
- What do you think the term “cross-bracing” means?
- How do you think a cross-bracing design helps buildings withstand earthquake damage?
After students share their responses, tell students, “Earthquake-proof buildings typically have cross-bracing which are made by two diagonals supports placed in an X manner that forms triangles, which are the strongest geometric shape. This design geometry is often seen in bracing on bridges, and it helps keep the building steady by providing lateral stability. Cross-bracing supports and balances tension and compression forces to help prevent the structure from collapsing during an earthquake.”
- Now, based on the results of their first prototype and applying the above structural design tips, have students iterate (improve) on their original structure by redesigning and rebuilding a second prototype that is even more earthquake-proof. If needed, resupply students with replacement materials. For students that had a successful initial design, challenge them to add a third level to their model.
- Once students have designed and built their second building prototype, repeat steps 6 and 7.
- Have a class discussion on the results of their second prototypes. Which design elements were most effective at withstanding the earthquake forces and which parts failed. Reinforce that failing and learning from failure are keys to success in engineering, and that failure is not “bad” or “wrong” but valuable information. Ask students if they had more time and materials how would they improve their next model.
- Have students reflect about the science phenomena they explored and the science and engineering skills they used today by completing the Making Sense Assessment.
Assessment
Pre-Activity Assessment
Discussion: Discuss with students how natural processes create earthquake hazards. Teachers can also use the Earthquakes Rock! lesson to demonstrate how earthquakes cause waves of movement in the ground.
T-Charts: Have students make T-charts as described in the Introduction/Motivation section.
Activity Embedded Assessment
Measurement: Have students measure the length, width, and height of their structures and calculate the volume using the equation V = L x W x H.
Before/After: Have students list types of changes they expect to see in their structures before they test them, then illustrate changes in their structures, after they complete the simulations. Ask students to identify one variable and discuss what happened when they changed the variable. They could reflect on these changes and their causes in a post-activity science journal entry.
As another option, show students before and after pictures of earthquake damage, comparing buildings which remained standing with the least damage to those which did not survive. Students could discuss possible reasons for the damage, observing structure (height, etc.) of the buildings.
Post-Activity Assessment
Drawing the Geometry: Have students make drawings and label the shapes in their designs (cube, pyramid, triangle, etc.).
Making Sense: Have students reflect about the science phenomena they explored and/or the science and engineering skills they used by completing the Making Sense Assessment.
Safety Issues
Inform students that in an experimental testing lab or during science experiments, nothing should ever be put into their mouths. The marshmallows and Jell-O® are not for consumption. Instead, set some aside for a treat after the activity.
Troubleshooting Tips
The activity works best with fresh (soft) marshmallows. As the marshmallows sit out and dry out, they and the structures become stable and rigid.
Do not leave the Jell-O® uncovered too long, as it dries out and becomes less fluid, which affects the activity results.
Activity Extensions
Have students make observations about buildings in the community or a nearby city. Ask students to observe the design of the buildings and whether they appear to be earthquake-proof.
Research how to obtain fault maps of the area. The U.S. Geological Survey (https://www.usgs.gov) or the Federal Emergency Management Agency (https://www.fema.gov) are good places to search. Is the area in a zone at risk for earthquakes? Do local building codes and architecture plan for this?
Activity Scaling
For higher grades, give students more requirements and constraints for their model buildings.
Subscribe
Get the inside scoop on all things TeachEngineering such as new site features, curriculum updates, video releases, and more by signing up for our newsletter!More Curriculum Like This

Students learn about the types of seismic waves produced by earthquakes and how they move through the Earth. Students learn how engineers build shake tables that simulate the ground motions of the Earth caused by seismic waves in order to test the seismic performance of buildings.

They make a model of a seismograph—a measuring device that records an earthquake on a seismogram. Students also investigate which structural designs are most likely to survive an earthquake.

Students learn about tsunamis, discovering what causes them and what makes them so dangerous. They learn that engineers design detection and warning equipment, as well as structures that that can survive the strong wave forces. In a hands-on activity, students use a table-top-sized tsunami generator...
References
http://earthquake.usgs.gov/4kids/
Copyright
© 2004 by Regents of the University of ColoradoContributors
Jessica Todd; Melissa Straten; Malinda Schaefer Zarske; Janet YowellSupporting Program
Integrated Teaching and Learning Program, College of Engineering, University of Colorado BoulderAcknowledgements
The contents of this digital library curriculum were developed under grants from the Fund for the Improvement of Postsecondary Education (FIPSE), U.S. Department of Education, and National Science Foundation (GK-12 grant no 0338326). However, these contents do not necessarily represent the policies of the Department of Education or National Science Foundation, and you should not assume endorsement by the federal government.
Last modified: July 27, 2023
User Comments & Tips