Quick Look
Grade Level: 11 (10-12)
Time Required: 3 hours 45 minutes
There are six sections of the activity over six days: Part One (70 minutes), Part Two (60 minutes), Part Three (15 minutes), Part Four (15 minutes), Part Five (30 minutes) and Part Six (40 minutes).
Expendable Cost/Group: US $0.00 This activity also uses non-expendable, or reusable, items; see the Materials List for details.)
Group Size: 4
Activity Dependency: None
Subject Areas: Physics, Problem Solving, Science and Technology
NGSS Performance Expectations:
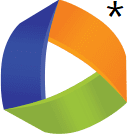
HS-PS4-5 |
Summary
Students take an illuminance reading of a light, find a way to approximate it at as an intensity reading, and then calculate the photon flux and radiation pressure while assessing the precision of these values. The ideas are then applied to speculate about engineering challenges involved in using ground-based lasers to propel interstellar spacecraft. In the process students must work through challenging problem solving and analyze the confidence in their results. There is also emphasis on comparing and contrasting the scientific method to the engineering design process.Engineering Connection
Light exerts force so it can accelerate things, even spacecraft. Engineers at the Breakthrough Starshot project are tackling a host of engineering problems to use lasers to send spacecraft to one of the closest stars outside our Solar System, Alpha Centauri. Students are given the task of engineering a viable process for converting from illuminance to intensity. After reflecting on the role of engineering in that work, they pick some of the problems engineers on the Breakthrough Starshot project wrestle with, like how to hit a tiny spacecraft in orbit with a laser fired from the ground, or how to keep the mass of the spacecraft low while allowing it to carry the instrumentation needed to explore the Alpha Centauri system. Students then brainstorm their own solutions.
Learning Objectives
After this activity, students should be able to:
- Demonstrate resourcefulness when working on challenging, open-ended questions.
- Calculate radiation pressure and photon flux from electromagnetic intensity.
- Compare and contrast the scientific method to the engineering design process.
- Use rounding to express a numerical result with optimal confidence.
Educational Standards
Each TeachEngineering lesson or activity is correlated to one or more K-12 science,
technology, engineering or math (STEM) educational standards.
All 100,000+ K-12 STEM standards covered in TeachEngineering are collected, maintained and packaged by the Achievement Standards Network (ASN),
a project of D2L (www.achievementstandards.org).
In the ASN, standards are hierarchically structured: first by source; e.g., by state; within source by type; e.g., science or mathematics;
within type by subtype, then by grade, etc.
Each TeachEngineering lesson or activity is correlated to one or more K-12 science, technology, engineering or math (STEM) educational standards.
All 100,000+ K-12 STEM standards covered in TeachEngineering are collected, maintained and packaged by the Achievement Standards Network (ASN), a project of D2L (www.achievementstandards.org).
In the ASN, standards are hierarchically structured: first by source; e.g., by state; within source by type; e.g., science or mathematics; within type by subtype, then by grade, etc.
NGSS: Next Generation Science Standards - Science
-
Use mathematical representations of phenomena or design solutions to describe and/or support claims and/or explanations.
(Grades 9 - 12)
More Details
Do you agree with this alignment?
NGSS Performance Expectation | ||
---|---|---|
HS-PS4-5. Communicate technical information about how some technological devices use the principles of wave behavior and wave interactions with matter to transmit and capture information and energy. (Grades 9 - 12) Do you agree with this alignment? |
||
Click to view other curriculum aligned to this Performance Expectation | ||
This activity focuses on the following Three Dimensional Learning aspects of NGSS: | ||
Science & Engineering Practices | Disciplinary Core Ideas | Crosscutting Concepts |
Communicate technical information or ideas (e.g. about phenomena and/or the process of development and the design and performance of a proposed process or system) in multiple formats (including orally, graphically, textually, and mathematically). Alignment agreement: | Solar cells are human-made devices that likewise capture the sun's energy and produce electrical energy. Alignment agreement: Information can be digitized (e.g., a picture stored as the values of an array of pixels); in this form, it can be stored reliably in computer memory and sent over long distances as a series of wave pulses.Alignment agreement: Photoelectric materials emit electrons when they absorb light of a high-enough frequency.Alignment agreement: Multiple technologies based on the understanding of waves and their interactions with matter are part of everyday experiences in the modern world (e.g., medical imaging, communications, scanners) and in scientific research. They are essential tools for producing, transmitting, and capturing signals and for storing and interpreting the information contained in them.Alignment agreement: | Systems can be designed to cause a desired effect. Alignment agreement: Science and engineering complement each other in the cycle known as research and development (R&D).Alignment agreement: Modern civilization depends on major technological systems.Alignment agreement: |
Common Core State Standards - Math
-
Reason abstractly and quantitatively.
(Grades
K -
12)
More Details
Do you agree with this alignment?
-
Choose a level of accuracy appropriate to limitations on measurement when reporting quantities.
(Grades
9 -
12)
More Details
Do you agree with this alignment?
-
Reason quantitatively and use units to solve problems.
(Grades
9 -
12)
More Details
Do you agree with this alignment?
-
Use units as a way to understand problems and to guide the solution of multi-step problems; choose and interpret units consistently in formulas; choose and interpret the scale and the origin in graphs and data displays.
(Grades
9 -
12)
More Details
Do you agree with this alignment?
-
Solve equations and inequalities in one variable
(Grades
9 -
12)
More Details
Do you agree with this alignment?
International Technology and Engineering Educators Association - Technology
-
Students will develop an understanding of the attributes of design.
(Grades
K -
12)
More Details
Do you agree with this alignment?
-
Students will develop an understanding of engineering design.
(Grades
K -
12)
More Details
Do you agree with this alignment?
-
Use computers and calculators to access, retrieve, organize, process, maintain, interpret, and evaluate data and information in order to communicate.
(Grades
9 -
12)
More Details
Do you agree with this alignment?
-
Illustrate principles, elements, and factors of design.
(Grades
9 -
12)
More Details
Do you agree with this alignment?
-
Use various approaches to communicate processes and procedures for using, maintaining, and assessing technological products and systems.
(Grades
9 -
12)
More Details
Do you agree with this alignment?
-
Synthesize data and analyze trends to make decisions about technological products, systems, or processes.
(Grades
9 -
12)
More Details
Do you agree with this alignment?
State Standards
New York - Math
-
Reason abstractly and quantitatively.
(Grades
Pre-K -
12)
More Details
Do you agree with this alignment?
-
Choose a level of accuracy appropriate to limitations on measurement when reporting quantities.
(Grades
9 -
12)
More Details
Do you agree with this alignment?
-
Reason quantitatively and use units to solve problems.
(Grades
9 -
12)
More Details
Do you agree with this alignment?
-
Use units as a way to understand problems and to guide the solution of multi-step problems; choose and interpret units consistently in formulas; choose and interpret the scale and the origin in graphs and data displays.
(Grades
9 -
12)
More Details
Do you agree with this alignment?
-
Solve equations and inequalities in one variable
(Grades
9 -
12)
More Details
Do you agree with this alignment?
New York - Science
-
Communicate technical information about how some technological devices use the principles of wave behavior and wave interactions with matter to transmit and capture information and energy.
(Grades
9 -
12)
More Details
Do you agree with this alignment?
Materials List
Each group needs:
- metric ruler
- Vernier Light Sensor or other light meter that reads in lux
- Vernier SpectroVis or other spectrophotometer
- Vernier Spectrophotometer Optical Fiber if the SpectroVis is used
- Vernier interface if Vernier sensors are used
- LED Light Source (such as a flashlight or desk lamp)
- Access to a computer with internet
- Copies of attachments (one per student unless otherwise noted):
- Part 1: Interpreting the Lux Reading
- Part 1: Converting from Lumens to Watts – one per group
- Part 4: Comparing Science and Engineering Methods
- Part 5: Breakthrough Starshot Project
- Part 6: Comparing Science and Engineering Methods II
Worksheets and Attachments
Visit [www.teachengineering.org/activities/view/ind-2350-radiation-pressure-photons-intensity-flux] to print or download.Pre-Req Knowledge
Students should know that:
- Electromagnetic radiation is quantized.
- Photon wavelength is related to energy and momentum by these equations:
- E = hc/λ
- p=E/c
- p=h/λ
- The impulse/change of momentum relationship.
- Power is the rate at which energy is produced, transmitted, received, etc.
- Pressure is force applied per area.
- The rules of significant figures.
- The meaning of order of magnitude.
Introduction/Motivation
In the physical sciences we describe the strength of light in terms of how much energy lands on a surface (or passes through a given plane) over time. This is usually expressed in units of watts per square meter and is called the intensity of the light. In general, the measurement of electromagnetic radiation is called radiometry.
There is also the related field of photometrics, which is the measure of how bright light appears to our eyes. Apparent brightness (measured in lux) is important in photography and in lighting design.
Most light meters you encounter make photometric measurements. However, can we do the same by measuring lux? Can lux be converted into watts per square meter? In theory, yes, but because the eye sees different wavelengths as having different brightness when each has the same intensity, this is a difficult conversion to make with white light. Still, if you are clever and resourceful, you can figure out how to do the conversion approximately and express the accuracy of that approximation.
The advantage of working with watts per square meter rather that lux is that many engineering applications are based on the actual levels of energy expressed by watts. An obvious connection with light energy is the design and placement of solar panels. A less common application is using light to propel spacecraft. NASA’s Nanosail D mission, launched in 2010, was the first successful test of this technology. Light sails are the working model for the proposed Breakthrough Starshot to Alpha Centauri. Light sails were also in the news in 2018 when calculations by researchers at the Harvard Smithsonian Center for Astrophysics led to the suggestion that the ‘Oumuamua asteroid that passed through our solar system might have been a light sail on an alien craft.
Procedure
Background
It will help students if they are fluent in discussing the shifts between wave and particle descriptions of light. The wave model describes light in terms of wavelength and frequency, and energy can have any value depending on the source and the duration of the wave. The particle models refer to quanta, or photons of light. The term “photon” is solely reserved for visible light whereas “quantum” can be applied to any electromagnetic radiation, although the two terms are often used interchangeably in popular science.
This work uses the following equations:
- E = hc/λ
- p=E/c
- p=h/λ
The first of those equations can be thought as a tool for shifting between the particle and wave models of light. Light of wavelength λ can be viewed a stream of photons, each of energy E. Planck’s constant is represented by h (h = 6.626 x 10-34 J/s) and the speed of light in a vacuum by c (c = 2.998 x 108 m/s) in that equation. Once students find a way to approximate an illuminance reading as an intensity reading, this equation can estimate the number of photons.
The second equation relates the momentum p of a photon of light to the photon’s energy E for light of wavelength λ. The final equation similarly defines momentum, but in terms of the Planck’s constant and the wavelength of the light in the wave model.
How bright light appears to the human eye depends on the wavelength of the light. This relationship is represented numerically in the Converting from Lumens to Watts Sheet: the greater the lumen/watt ratio, the more sensitive the human eye is to that wavelength. The table shows the conversions for photopic settings (for lighting conditions under daylight) versus scotopic (for conditions in low-light) settings. Lumens measure the rate of light passing through a volume, adjusted for human vision. Illuminance is the number of lux falling per square meter of a surface.
The objective equivalent of illuminance is illumination. It is amount of light energy arriving per unit time on a given area. We usually express this as watts per square meter.
Before the Activity
- If necessary, instruct students how to set up the light meter and take a reading with it.
- The teacher should read through the Teacher Notes for The Feel of Photons.
- Make copies of the assessment documents and worksheets (one per student unless otherwise noted):
- Pre-Quiz
- Part 1: Interpreting the Lux Reading
- Part 1: Converting from Lumens to Watts – one per group
- Part 4: Comparing Science and Engineering Methods
- Part 5: Breakthrough Starshot Project
- Part 6: Comparing Science and Engineering Methods II
- Post-Quiz
- Prior to Part 5, ensure there is a method to show a short video to students.
With the Students
Part One: Taking and interpreting the Lux Reading
- Give the Pre-Quiz and collect it after students are finished. Comments on the goals of each question and the nature of answers student may submit are given in the Teacher Notes for The Feel of Photons. That document also includes tips and guidelines for the teachers on many other parts of this exercise.
- Ask students to work in groups and take a reading of a light source with a light meter that is 10 centimeters from the source. (It might be that your light is so bright it overwhelms the sensor at that range. If so, choose a greater distance. Ensure that students control the resulting distance so data can be shared and compared among groups can be compared.)
- Ask each group to report their readings. Lead a discussion on the range in values and frame it in terms of significant figures. Do we expect the readings to all be the same? Are the values in agreement to three significant figures? To two? (The emphasis here is on reminding students of how significant figures allow us to make statements about precision.)
- Give each student the Interpreting the Lux Reading to complete. Lead a discussion to compare responses from the entire class.
- Say the following to students:
As you know, the particle model of light tells us that a photon has a momentum equal to the amount of light energy divided by the speed of light. Based on that knowledge, we can determine how much momentum your light has and how hard the light pushes on a surface.
You can see that is not too hard if you know how much light energy comes from the LED source. The light meter, however, gave you a value in lux.
How can we calculate how many watts are in a lumen? Lumens are a matter of the subjective experience of brightness. Our eyes are more sensitive to some wavelengths than others. Under normal lighting conditions we best see light in the vicinity of 555 nm (yellow-green, as is sometimes seen in school busses and emergency vehicles). In low light conditions, we are most sensitive to wavelengths near 507 nm (blue-green, and this is related to why the night sky looks blue to us).
Distribute the Converting from Lumens to Watts Sheet. Instead of providing an explanation, have you students realize that doing the conversion requires knowing what wavelengths are present at what relative levels. Tell them you have a SpectroVis to help with that.
Alternatively, say to them:
If you had a single wavelength of light coming from the lamp, the conversion would be easy. The LED is white light, meaning it is many wavelengths mixed together. Fortunately, we have a tool to measure the relative intensities of the wavelengths in the light.
If the question arises, explain that the SpectroVis obtains the spectrum by putting the light through a diffraction grating that spreads it out by wavelength. The separated wavelengths fall onto a charged couple device (similar to a digital camera) that measures the relative energy of each wavelength. This is also explained on the SpectroVis product page.
Part Two: Figuring out how to convert from lumens to watts, and watts to momentum, number of photons and radiation pressure.
- Ask students to use the SpectroVis and Fiber Optic Sheet to get a measurement of the spectrum of the light source. It will look something like Figure 1.
Say to the students: Now you have a measurement of what wavelengths are present. The units of the vertical axis are “relative” because the SpectroVis is calibrated for wavelength but not for intensity. Still, we can use it to see which wavelengths are strong and which are weak. Knowing the lumen/watt ratio by frequency, you can get a ballpark value of the intensity.
There are several ways to approach this, but it will require some speculation, some approximation, and some deductive reasoning. There are ways to do that with just the materials you currently have. Feel free, however, to look up whatever you need online. Your answer will be an estimate, but with care and thought you can influence how good an estimate it is.
- Once students work out a value for the intensity of the light, ask them to calculate the momentum of the energy coming from the LED source. Allow students to see that this is a straightforward calculation using the relationship E=pc.
- Ask groups to estimate the photon flux. Again, let students work out on their own that this is done by dividing the intensity of the source by the energy of the photon. That will require an assumption about the wavelength of the light. If students solved the lumen-watt conversion using the SpectroVis, they will already have a method for approximating the wavelength or wavelengths to use. They otherwise need to work out one or more representative wavelengths to use in the calculation. Doing that will likely lead them through an analysis similar to that used to find the total intensity.
- Ask groups to determine the force that the light applies to a surface. The basic reasoning is that force is the rate of change of momentum. Students have the data needed to find the force applied per area.
Part Three: Assessing confidence in the results
- Have students discuss in groups about how confident there are in their results. Remind them that it is “not whether it is true or false but rather how likely it is to be true or false” and that it is reasonable to take into account the values for intensity and momentum from the other groups.
- Instruct students to try to come up with a statement about the results that they are confident about, but without being so vague that it understates the strength of their conclusion. For example, if a student is highly confident that more than millions of photons land per second, but that they can say something much more precise than that.
- A good way to approach this is to write several possible “approximate statements with different degrees of certainty”, put them in order of their confidence in them. Students can then choose the one that strikes the best balance between confidence and precision.
- Assign each student the task of formally writing up some portion of this work. It might be just one of the four calculations (the conversion of the measured illuminance to intensity, momentum of the light, the radiation pressure or the photon flux). It could be combination of those. The writing will serve as an assessment of progress toward the learning objectives.
Part Four: Introducing the Engineering Design Process
- Ask students to complete the exercise in the Comparing Science and Engineering Methods Handout and turn it in.
Part Five: Breakthrough Starshot Project
- Give students the Breakthrough Starshot Program Sheet to do in groups.
- Ask each group to present the ideas it develops to the class.
- After the presentations, debrief with the video “Will Starshot’s Interstellar Journey Succeed?” by PBS Space Time for the main considerations of Breakthrough Starshot.
Part Six: Wrapping up
- Give students another copy of the Comparing Science and Engineering Methods II Handout to do individually.
- Give students the Post-Quiz to do individually.
Vocabulary/Definitions
illuminance: A measure of how much light falls on a surface, adjusted for the fact that humans perceive different wavelengths more or less readily.
intensity: The amount of energy incident per unit time on an area.
lumen: A unit for the rate at which light energy, when adjusted for human perception, arrives on a surface.
lux: One lumen per square meter.
photon flux: The number of photons landing in an area per unit time.
photopic: Relating to or denoting vision in daylight or other bright light.
radiation pressure: The force per area applied by electromagnetic radiation.
scotopic: Relating to or denoting vision in dim light.
Assessment
Pre-Activity Assessment
Pre-Quiz: Conduct the Pre-Quiz. The activity starts with five questions, the first two of which ask students to modify a drawing to represent how they envision the role of light in looking at a light source. The other questions check student understanding of differing conventions for stating power of light bulbs and lasers and establish a baseline for their thinking about the relationship between science and engineering.
Activity Embedded Assessment
Formative Assessment of Resourcefulness: Students are assessed through observation while they work on challenging, open-ended questions in all parts of this activity. The Formative Assessment of Resourcefulness attachment sketches out a framework for making and interpreting those observations.
Post-Activity Assessment
Post-Quiz: Have students complete the Post-Quiz. The final step of the activity asks two questions: 1) identify how the students think about the relationship between sciences, and 2) how they think about the precision of the value they got for the photon flux.
Activity Extensions
1) The Feasibility of Light Drives for Spacecraft Extension sets students up to brainstorm and conduct internet research to probably lead them to identify practical problems with getting thrust from a laser on board a vessel. If so, you can let students take a supplemental pass at comparing the scientific method and the engineering design process.
The question asked in the exercise is clearly an open-ended. The handout raises the matter of the mass of the probe, and provides a range of manned and unmanned probe masses to save students some trouble.
Another consideration will likely be the power and mass of lasers. Do not deter students from looking up how powerful lasers can be. You may need to emphasize for them that the power of a laser (contrary to the convention for light bulbs) refers to the output.
Mass data for lasers is not readily available in most cases, but something might be gleaned from the items on the following list. You may want to provide them with this information or suggest to students that searching for lasers as weapons might be productive, since having powerful, portable lasers is of interest to the military.
- Boeing makes a 10 kW laser weapon small enough that can be mounted on a large airplane.
- Raytheon hopes to make a 25 kW laser small enough to fit on a Humvee.
- There is industry speculation that engineers at Northrop Grumman are developing a 30 kW laser would be mounted on a tank.
- Kratos has built a 33 kW laser that needs a warship to accommodate it.
- LFEX, or Laser for Fast Ignition Experiments, measures more than 300 feet long.
- Osaka University has fired a 2 pettawatt (2 x 1015 watt) laser, but only for 10-12 seconds. (Consider as an extension watching that video and assessing the claims that “In 2010 the entire world’s population used an estimate 16 terawatts of electricity. It takes 1000 terawatts to make one petawatt. That means this laser’s output represented more than one hundred times the amount of electrical power consumed by the entire world in 2010”. The speaker goes on to clarify that the laser fires briefly, but he is still confusing rate of energy consumption with an amount of energy.)
Students will likely see that the low thrust and high mass of powerful lasers make using a laser on a spacecraft a poor propulsion choice. Some may get the idea (or have heard from other sources) that a laser on the ground could fire up to orbit and push a spacecraft.
Some other considerations:
- The thrust will be small. (Ion propulsion has that same issue, yet was used on Deep Space 1, so small thrust is not a deal-breaker.)
- Travel will take a long time.
- Some missions can afford to take a long time, especially if it lowers costs.
- Efficiency of energy conversion from power source to propulsion impacts cost.
- The feasibility question is related to whether the method used proven technologies used or technologies that need to be developed.
- Propelling he probes with ground-based lasers requires compensating for the Earth rotating under the probes.
- The probes have no way of slowing when they arrive at their target.
2) Students can conduct Internet research to investigate why the eye has peak sensitivity to 555 nm light under photopic conditions but to 527 nm light under scotopic conditions.
3) Students can conduct internet research to investigate whether animals have peak sensitivities to light wavelengths that differ from humans.
Activity Scaling
- For lower grades, lay out the different methods for converting illuminance to intensity described in Teacher Notes for The Feel of Photons attachment under the “Supporting students converting the lumens to total energy” heading.
- Ask different groups to use different methods, comparing results at the end. For the Breakthrough Starshot portion of the work,
- Give students the chart showing atmospheric absorption by wavelength and ask what wavelengths are best to use.
- Give several of the factors listed in Teacher Notes for The Feel of Photons on this portion of the work and ask the students rank them from most to least significant.
Subscribe
Get the inside scoop on all things TeachEngineering such as new site features, curriculum updates, video releases, and more by signing up for our newsletter!Copyright
© 2020 by Regents of the University of Colorado; original © 2018 Jonathan HeilesContributors
Jonathan HeilesSupporting Program
Jonathan Heiles, Poughkeepsie Day School, New York, USAAcknowledgements
The author expresses special thanks to Dr. Casimer DeCusatis, Marist College Department of Computer Science, Poughkeepsie, NY, for general support and for comments on earlier drafts of this work.
Last modified: July 21, 2023
User Comments & Tips